人类肿瘤中BRAF改变的叙述性综述:诊断和预测意义
引言
B-Raf原癌基因,丝氨酸/苏氨酸激酶(BRAF)是人类肿瘤发生过程中最常见、最具特征性的癌基因之一。携带BRAF突变的肿瘤谱系基本上横跨每个器官系统,既代表惰性肿瘤,也代表高度侵袭性恶性肿瘤。临床测序技术的进步使得常规检测V600E热点以外的BRAF改变成为可能,从而产生了新的临床病理相关性。针对RAF和MEK通路激活的靶向治疗的发展催生了许多BRAF生物标记物驱动的临床试验,首先是针对黑色素瘤的BRAF抑制剂单一治疗,随后是探索性泛癌症篮子试验,最后是目前被批准用于晚期或转移性BRAF V600E突变肿瘤患者的特异性靶向抑制剂联合治疗。了解BRAF改变的频谱和靶向性现在是诊断和治疗肿瘤学实践的基础。本文将综述BRAF改变在肿瘤发生中的作用,检查最近定义的与下游信号转导和靶向性有关的BRAF改变的类别,回顾在临床实践中选择BRAF靶向实例,并讨论检测BRAF突变的分子诊断。我们根据叙述性综述检查表(可在https://pcm.amegroups.com/article/view/5933/rc)呈现下文。
方法
本文使用的文献摘自国家医学图书馆的PubMed.gov,包括1997至2020年间以英语发表的全文手稿。必要时,通过对其申办网站组织进行有针对性的检索来识别会议摘要,通过谷歌检索识别监管文件。
BRAF结构与功能
BRAF属于丝氨酸/苏氨酸激酶快速加速型纤维肉瘤(RAF)家族,在MAP激酶/ERK信号级联中作为丝裂原活化途径激酶(MAPKKK)发挥作用。BRAF通常在配体与受体酪氨酸激酶(RTK)如表皮生长因子受体(EGFR)或ERBB2结合后被触发。RTK磷酸化导致Ras家族GTPases的激活,从而触发RAF家族成员的二聚化和下游激酶的激活,包括MEK1/2和ERK1/2,导致直接和间接的转录调控,参与细胞的生存和增殖[1]。已经描述了三个不同的Raf基因,即ARAF、BRAF和CRAF(RAF-1);所有这些基因都被证明在发育和肿瘤发生中发挥重要作用。然而,BRAF最大限度地被致癌的RAS信号激活,而ARAF和CRAF似乎需要依赖于Raf的酪氨酸磷酸化[2]。BRAF位于染色体7q34上,由18个外显子组成,包含3个在Raf家族成员中保守的区域:C1,包含Raf样Ras结合结构域和激酶结构域的自身抑制因子(由氨基酸150-290编码);C2,包含富含丝氨酸和苏氨酸的铰链区(由氨基酸360-375编码);以及C3,包含蛋白酪氨酸激酶结构域(由氨基酸457-717编码)。
疾病中的BRAF
位于C1结构域和蛋白酪氨酸激酶结构域内的种系BRAF突变可能引起心-面-皮肤综合征,其与面部畸形、精神发育迟滞和心脏缺陷相关。患有这种疾病的个体很少继续发展为恶性肿瘤[3,4]。而BRAF的体细胞突变是人类报告的最常见的致癌改变之一,可在成人和儿童癌症患者、实体瘤和液体肿瘤中发现,并作为高度侵袭性和惰性肿瘤的明显驱动因素。在6%的人类恶性肿瘤中总体报告了BRAF的致癌突变,主要位于C3区域内[1]。尽管BRAF Val600Glu(V600E)突变是诊断和治疗目的中最公认的突变,但已报告该基因中超过200种致癌改变,对下游通路激活和靶向性具有一系列影响[5]。
致癌与衰老的BRAF突变在2002年首次被认为是致癌的,当时参与癌症基因组计划的研究人员描述了一系列癌症类型中的V600E突变(当时被注释为V599E),包括三分之二的黑色素瘤[6]。然而,在超过80%的良性痣中也发现了这些相同的突变,这表明BRAF V600E不足以推动肿瘤的发生[7]。在良性克隆过程中,如nevi,BRAF癌基因驱动的衰老通过诱导肿瘤抑制因子p16INK4a和稳定的细胞周期阻滞来阻止细胞增殖[7,8]。绝大多数良性痣未能进展为恶性,有些甚至可能消退。相反,在黑色素瘤中,前体细胞似乎首先获得肿瘤抑制基因,如CDKN2A和/或PTEN;在这种情况下,MAPK基因的后续突变,包括BRAF,可以推动恶性转化[9]。事实上,似乎在大多数BRAF突变复发的肿瘤环境中,转化需要多种肿瘤抑制基因的失活。癌基因诱导的衰老也与端粒功能障碍有关;因此,在一组肿瘤中,hTERT基因表达的下调,例如通过TERT启动子突变,可以恢复端粒酶活性并逃避衰老[10]。
除了黑色素细胞痣,BRAF基因突变还与广泛的良性增生和低恶性潜能的肿瘤有关,这些肿瘤影响到身体的大部分器官。这些肿瘤包括输卵管内膜病(11例)、后肾腺瘤(12例)和后肾间质瘤(12例)、乳头状颅咽管瘤(13例)、神经节胶质瘤(14例)、垂体腺瘤(15例)、支气管腺瘤/纤毛化多结节乳头状瘤(16,17例)、ErdheimChester病(18例)、朗格汉斯细胞组织细胞增生症(19例)和无柄锯齿状腺瘤。含有BRAF V600E突变的惰性或低级别肿瘤的例子将在下面更详细地描述。
肿瘤发生与衰老
BRAF突变在2002年首次被认为是致癌性的,当时参与癌症基因组计划的研究者描述了包括2/3黑色素瘤在内的一系列癌症类型中的V600E突变(当时注释为V599E)[6]。然而,在超过80%的良性痣中也描述了这些相同的突变,表明BRAF V600E不足以驱动肿瘤发生[7]。在良性克隆过程中,如痣,BRAF肿瘤发生驱动的衰老通过诱导肿瘤抑制因子p16INK4a和稳定的细胞周期停滞阻断细胞增殖[7,8]。绝大多数良性痣不会进展为恶性肿瘤,有的甚至可消退。相反,在黑色素瘤中,前体细胞似乎首先获得CDKN2A和/或PTEN等肿瘤抑制基因的命中;在这种情况下,包括BRAF在内的MAPK基因的后续突变可以驱动恶变[9]。事实上,在大多数BRAF突变复发的肿瘤背景下,多种肿瘤抑制基因的失活似乎是转化所必需的。癌基因诱导的衰老也与端粒功能障碍有关;因此在肿瘤的一个亚群中,hTERT基因表达的去阻遏,如通过TERT启动子突变,恢复端粒酶活性并逃避衰老[10]。
除黑素细胞痣外,BRAF突变还与影响身体大多数器官的广泛良性增殖和低恶性潜能肿瘤相关。包括输卵管内膜异位症[11]、后肾腺瘤[12]和后肾间质瘤[12]、乳头状颅咽管瘤[13]、神经节胶质瘤[14]、垂体腺瘤[15]、支气管腺瘤/肺纤毛结节状乳头状瘤[16,17]、Erdheim-Chester病[18]、朗格汉斯细胞组织细胞增生症[19]和结肠无蒂锯齿状腺瘤[20]。已选择含有BRAF V600E突变的惰性或低级别肿瘤的例子将在下面更详细地描述。
输卵管内膜异位症
输卵管内膜异位症定义为存在形态学上良性的腺体结构,包括输卵管上皮累及腹膜或淋巴结,被认为是卵巢低度恶性浆液性肿瘤的前兆。因此,在两种病变中均发现了相似的驱动致癌事件,在输卵管内膜异位症和低度浆液性肿瘤患者中,在两个人群中均可检测到常见的KRAS G12/G13或BRAF V600E突变[11]。在输卵管或卵巢表面上皮细胞的细胞培养模型中,突变型KRAS或BRAF的存在触发生长停滞[11,21];与该观察结果一致,输卵管内膜异位症的增殖率较低。肿瘤抑制通路的额外缺陷可能是驱动从输卵管病向临床可检测的浆液性肿瘤演变的必要条件。
组织细胞增生症
组织细胞增生症(ECD)和朗格汉斯细胞组织细胞增生症(LCH)是累及儿童和成人的进行性、全身性肿瘤过程,目前世界卫生组织将其描述为炎性髓系肿瘤。ECD由CD68+组织细胞组成,而LCH由CD1a+ CD207+组织细胞组成;两者均与多器官受累相关。15%的ECD患者还患有LCH,这种情况被归类为“重叠组织细胞增多症”[22]。肺LCH与吸烟独特相关,症状性和放射学后遗症通常可仅通过戒烟进行管理。ECD和LCH的生物学相关性得到了相似基因组特征证据的部分支持,包括频繁的BRAF V600E突变(使用高灵敏度分子技术时分别约为70%和60%)和相互排斥的MAP2K1热点突变(分别为20%和12%)[22,23]。在一小部分LCH病例中报告了其他BRAF激活事件,包括插入缺失、重复或融合[24]。BRAF V600E突变最初被报道为组织细胞增多症的唯一改变[25]。然而,后来的研究报道了侵袭性病例中TP53的突变[26]或p16(INK4a)的缺失[27],这与至少两次打击的肿瘤发生模型以及肿瘤抑制功能受损以将BRAF突变的细胞从衰老中释放出来的需要一致。
结肠无蒂锯齿状腺瘤/息肉
BRAF V600E突变在60%~80%的锯齿状肿瘤中报告[28],但在传统结肠腺瘤中不存在。无蒂锯齿状腺瘤/息肉(SSA/P)被认为代表CpG岛启动子甲基化高/微卫星不稳定性高(MSI-H)的良性前体结肠癌症。因此,SSA/P显示BRAF V600E频繁突变,获得的WNT通路活性和广泛的CpG岛甲基化,包括MLH1启动子甲基化,在进展为细胞学异型增生的过程中[29,30]。少数BRAF突变的SSP/A可能通过获得包括TP53和PTEN在内的肿瘤抑制基因突变,沿着微卫星稳定途径进展[31]。
实体恶性肿瘤中发现的致癌改变类型概述
在一组不同的实体瘤中报告了激活体细胞BRAF改变,包括突变、融合和扩增。这些常见于原发性脑肿瘤,其次为非滤泡性甲状腺肿瘤、黑色素瘤和结直肠癌。其他实体瘤存在长尾,1%~5%的病例(肺腺癌、胰腺腺泡细胞癌、肝内胆管癌)或少于1%的病例(前列腺癌和膀胱癌、高级别浆液性卵巢癌)显示突变和/或融合[32](图1)。
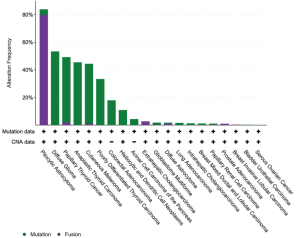
低分级中枢神经系统肿瘤的一个子集表现出特别高的BRAF激活改变的频率。BRAF V600E突变见于几乎所有的乳头状颅咽管瘤。在超过80%的病例中毛细胞型星形细胞瘤携带BRAF融合或突变,在高达50%和66%的病例中神经节胶质瘤和多形性黄色星形细胞瘤携带BRAF融合或突变。相反,BRAF突变在多形性胶质母细胞瘤中罕见。BRAF改变在低分级原发性脑肿瘤中具有不同且相互矛盾的预后意义;然而,确定这些变化可为BRAF靶向治疗的诊断和影响选择提供信息[33]。因此,分子分析和/或荧光原位杂交可常规用于临床实践,以检测这些变化并确诊形态学诊断。
BRAF突变的致癌活性
突变
致癌BRAF突变的特征是基于它们是否表现出激酶活性,需要上游RAS活性和BRAF二聚化。激酶激活突变与RAS信号传导无关。在这类突变中,一个子集信号为单体(1类),其他子集信号为组成性活性二聚体(2类)。BRAF V600E属于1类激活突变体,以及该密码子的其他不太常见的替换包括V600K/D/R/M。涉及V600密码子的其他小插入缺失突变罕见,但至少孤立的例子可能以与其他1类突变体相似的方式稳定活化的激酶[33]。至少在治疗前背景下,1类突变倾向于与其他致癌驱动改变(例如KRAS、EGFR、ALK融合等)以相互排斥的方式发生[34]。2类突变体聚集在密码子601、597、469和464[5]。最后,BRAF突变的一个子集表现出低至无激酶(激酶死亡)活性,下游信号的激活依赖于RAS活性。这些所谓的3类突变体分散在外显子11和15的热点中,代表了结直肠癌中观察到的高达30%的BRAF突变和非小细胞肺癌中观察到的高达30%的[1](图2),偶尔与RAS家族成员基因的致癌突变串联。机制上,这些RAS激活的激酶低/死亡突变体似乎与CRAF异二聚体化触发ERK信号,从而放大ERK通路的其他输入[5]。虽然1类(V600)突变体对靶向RAF抑制剂敏感,但2类突变体可能需要双重抑制RAF和下游MEK信号[36],3类突变体似乎对MEK抑制剂有反应[5]。
融合和其他结构变异
致癌性BRAF融合首次在甲状腺乳头状肿瘤中报告,富集于切尔诺贝利核事故后暴露于辐射的个体中[37]。AKAP9-BRAF是第一个具有特征性的融合基因,由AKAP9的前8个外显子和BRAF的C端部分(包括外显子9-18)组成,由7号染色体长臂上的旁着丝粒染色体倒位事件导致。该事件导致N端部分的CR1和CR2调节结构域缺失的BRAF,保留激酶结构域。与蛋白调控区的丢失一致,融合产物表现出组成性RAF激酶活化和转化能力。随后在毛细胞型星形细胞瘤中描述了结构相似的融合,其中7q34上的重复事件导致KIAA1549的N末端和BRAF的C末端从外显子9或11开始融合[38]。其他不太常见但复发的融合包括FAM131B-BRAF,由毛细胞型星形细胞瘤中7q[39]的间质缺失和肺腺癌和胰腺腺泡细胞癌中SND1-BRAF突变导致[40]。在一系列肿瘤中,包括毛细胞型星形细胞瘤、spitzoid黑色素瘤和其他频率极低的实体瘤类型,已在1~2份报告中确定了超过50个额外的融合伴侣[41,42]。在黑色素瘤和婴儿纤维肉瘤中很少报告BRAF激酶结构域的内部串联重复和/或N末端调节结构域的基因内缺失[43,44]。
融合事件被认为是2类改变;N-末端调节域的丢失使RAS不依赖于同源二聚化,这是这类突变体激活激酶所必需的。与这一类中的点突变一样,BRAF融合驱动MAPK通路信号传递,但对第一代BRAF抑制剂如vemurafenib具有耐药性[45]。抑制BRAF同源和异源二聚体的下一代RAF抑制剂在BRAF融合驱动的肿瘤中可能具有更高的活性[46]。
BRAF突变的治疗意义
黑色素瘤
在超过40%的皮肤黑色素瘤中报告了BRAF的致癌突变,在无慢性日光损伤的皮肤上发生的肿瘤中富集,并聚集在密码子V600及其周围[47]。最常见的替换是V600E,其次是V600K;其他替换包括V600R/M/D/G和该位置的小插入缺失并不常见[48]。维罗非尼单药治疗BRAF V600E/K突变显示客观缓解率约为50%[49-51]。在已发表的单药治疗试验中,与标准治疗相比,维罗非尼治疗改善了患者的总生存期[52],但受到耐药性发生的限制[53,54]。暴露于BRAF抑制剂的黑色素瘤采用异质性机制重新激活MAPK和替代通路[55]。与维罗非尼单药治疗相比,RAF和MEK联合治疗(达拉非尼、曲美替尼)改善了总生存期,缓解率>60%[56],但在BRAF抑制剂难治性黑色素瘤患者中,单药治疗后仅显示了适度的疗效[57]。目前(2020年)的临床试验和治疗指南建议对任何III期或IV期皮肤黑色素瘤患者进行BRAF突变检测;检测到BRAF V600激活突变证明使用BRAF-MEK治疗是合理的,包括达拉非尼/曲美替尼、维莫非尼/考比替尼或encorafenib/binimetinib。这些治疗可用于一线治疗或PD-1抑制剂免疫治疗进展后的治疗[58]。密码子L597和K601发生的突变也可以预测对MEK或MEK/RAF抑制剂联合治疗的反应,然而其他外显子11和15突变不能预测[36]。PD-1抑制剂pembrolizumab临床试验的事后分析证明了该免疫治疗的疗效,与BRAF V600突变状态或既往BRAF/MEK抑制剂治疗无关[59]。
靶向黑色素瘤以外的BRAF
在黑色素瘤BRAF抑制剂治疗的积极试验后,启动了篮子试验,招募各种BRAF V600突变的非黑色素瘤癌症患者,以确定靶向BRAF抑制剂是否可能是一种有前景的跨诊断方法。维罗非尼单药治疗的初步结果令人失望,然而,在NSCLC和组织细胞增多症中观察到大多数活性。在其他肿瘤类型(包括胆管癌、甲状腺未分化癌和卵巢癌)中偶尔观察到持久缓解,在结肠癌中联合维莫非尼和西妥昔单抗未观察到活性[60]。鉴于这些充其量是适度的初始结果,研究者开始在各种临床背景下寻找联合治疗。
结肠
10%的结直肠癌报告BRAF V600E突变,富集于右侧肿瘤和由于MLH1启动子甲基化导致的散发性错配修复缺陷的肿瘤,并作为选择接受抗EGFR(如西妥昔单抗)治疗的患者的排除标准。这是基于以下假设:RAF/RAS激酶成员(如KRAS、NRAS和BRAF)的激活导致组成性MAPK通路活性,与RTK信号转导无关,如通过EGFR[1]。在接受抗EGFR单克隆抗体治疗的结肠癌患者的回顾性研究中,BRAF V600突变作为缓解的阴性预测因子[61,62]。临床前研究证明,结肠癌中BRAF V600E的抑制导致EGFR的反馈激活和MAPK通路通过CRAF的旁路激活。事实上,抗BRAF和抗EGFR药物联合使用在杀死BRAF突变结肠癌细胞方面具有协同效应[63,64]。MEK和BRAF抑制剂联合治疗的Ⅰ/Ⅱ期研究显示,在BRAF V600E突变结肠癌患者中,与单独使用BRAF抑制剂相比,应答适度改善[65]。在BRAF V600E突变转移性结直肠癌(BEACON)患者中进行的三联BRAF、EGFR和MEK抑制试验证实了与标准治疗相比的耐受性和改善的疗效[66,67]。2018年,FDA授予BRAF、MEK和EGFR抑制剂组合(分别为encorafenib、binimetinib和cetuximab)作为二线治疗的突破性疗法认定[68]。在BEACON研究中,与标准治疗相比,在二线或三线治疗中接受encorafenib和西妥昔单抗双联治疗的患者也显示缓解率和生存期显著改善,导致2020年FDA批准[69]。非V600突变与抗EGFR治疗无效无明确相关性[70,71]。理论上,其他RAS通路成员缺乏共突变并保留对RTK信号传导依赖性的3类突变肿瘤子集可能从抗EGFR治疗中获益[72]。
60%的散发性MLH1启动子甲基化错配修复缺陷(MMR-D)/MSI-H结直肠癌具有BRAF V600E突变;这与仅约1%的生殖系MMR突变患者发生的结肠癌形成对比[73]。因此,对BRAF V600E状态的了解可提示Lynch综合征相关与散发性结直肠癌的可能性。BRAF突变与MMR/MSI状态一起,是结直肠癌公认的预后指标。MSI-H/BRAF野生型肿瘤的生存结局最好,而微卫星稳定(MSS)/BRAF突变肿瘤与不良预后相关。MSS/BRAF野生型肿瘤似乎具有中间结局[74,75]。除了与结肠癌中BRAF突变相关外,MMR-D状态还可预测对PD-1抑制剂治疗的反应性[76,77]。在一项比较PD-1抑制剂帕博利珠单抗与一线标准化疗治疗MMR-D/MSI-H结直肠癌患者的Ⅲ期试验中,与细胞毒性治疗相比,帕博利珠单抗使无进展生存期延长一倍以上,且重度不良反应减少[78]。这导致FDA批准帕博利珠单抗(pembrolizumab)作为MMR-D/MSI-H状态结直肠癌的一线治疗,不考虑BRAF或其他致癌驱动突变状态[79]。
肺
BRAF在NSCLC中表现出不同的突变;仅不到一半报告的致癌BRAF突变发生在V600E;其余主要分布在外显子11和15,与2类或3类活性相关(图2)。FDA已批准达拉非尼和曲美替尼(RAF和MEK抑制剂)联合治疗作为晚期/转移性BRAF V600E突变NSCLC患者的一线治疗[80]。与化疗相比,该组合为与缓解、无进展生存期和总生存期改善相关[81],一部分患者出现持久获益,超过1/3的接受一线治疗的患者存活超过3年[82]。这种联合治疗持久缓解可能与NSCLC基因组的简单性有关,更复杂的遗传变化和共突变预测获益持续时间较短[82]。BRAF V600E突变患者的临床特征具有异质性,包括从不吸烟者和既往/当前吸烟者。腺癌是观察到的最常见的组织学。与EGFR/ALK/ROS1改变的肺癌(往往对免疫治疗仅表现出非常有限的反应)相反,BRAF突变肿瘤(包括V600E和非V600E突变)确实表现出对免疫检查点抑制剂的反应[83]。根据临床前数据,RAF/MEK抑制剂联合治疗非V600E BRAF突变不被批准,这表明已批准的BRAF抑制剂对突变导致BRAF二聚化的肿瘤的疗效有限。在NSCLC中,2类和3类BRAF突变更可能与KRAS突变同时发生,似乎与更具侵袭性的疾病相关[35]。在这种情况下尝试了MEK和pan-RAF抑制剂的试验,但受到药物毒性的限制[84]。
其他罕见肿瘤类型
BRAF和MEK抑制剂联合治疗已被批准用于BRAF V600E突变的甲状腺未分化癌[85],该病约占甲状腺癌总数的1%~2%,在近50%的病例中含有这种突变。超过三分之二接受该方案治疗的BRAF突变甲状腺未分化癌患者缓解,12个月总生存率为80%,显著高于历史1年生存率20-40%[86]。
BRAF抑制剂维莫非尼于2017年获批用于BRAF V600突变的ECD,基于VE Basket试验的结果,该试验入组了22例成人ECD患者;超过一半的患者出现应答,并报告神经系统症状和疼痛改善[87,88]。在其他罕见肿瘤类型中报告了对BRAF抑制(伴或不伴MEK抑制)的应答,包括BRAF V600E突变的成釉细胞瘤、恶性血管球瘤、胆管癌、唾液腺腺癌和胶质母细胞瘤[89-91]。
BRAF突变检测的诊断工具
多种方法已被用于检测BRAF V600突变,重要的是要认识到不同策略的优势和不足。从2011年批准维罗非尼治疗BRAF V600突变的黑色素瘤开始,伴随诊断批准的趋势开始规定正式接受的临床突变检测方法。Roche cobas 4800 BRAF V600突变试验被批准作为维罗非尼单药治疗以及与cobimetinib联合治疗的体外诊断[92]。这种靶向PCR测定方法很快受到批评,因为相对于基于无偏倚测序的方法,其无法检测导致V600E/K/R突变的不太常见的二核苷酸替换,并且灵敏度次优[93]。同时,相对于Sanger测序和引物延伸/片段分析检测,高度标准化的cobas检测似乎产生更少的无效结果[94],对于突变等位基因分数低于25%的样本,显示出优于Sanger测序的灵敏度[95]。临床实验室伴随诊断使用的一个实际缺点是获得所需的资本支出用于特定的平台仪器,为预算更严格的小型实验室的实施创造了障碍。许多分子实验室已经有适合分析特定临床相关突变的仪器,很少有动力购买另一件潜在重复的设备。使这一空间进一步复杂化的是,FDA最近批准了一种不同的BRAF检测方法——therascreen BRAF V600E Rotor-Gene QPCR试剂盒,需要专门的仪器——用于选择BRAF和EGFR抑制剂治疗的结直肠癌患者[96]。许多分子诊断专家会问,为什么需要两个不同的FDA批准平台来生成关于相同突变变化的信息。
2011—2015年超过1000项国家熟练度检测结果的分析表明,在依赖实验室开发检测的实验室中,96.6%的BRAF结果产生了可接受(正确)的缓解,而FDA批准的伴随诊断(包括Roche cobas BRAF和bioMerieux THxID BRAF检测)为93%;较低的性能是由Roche检测驱动的,并归因于其检测c.1798_1799delGTinsAA(p.V600K)二核苷酸替代的相对不灵敏度[97]。在另一项检查2014-2017年使用下一代测序与非NGS实践进行突变检测的能力检测分析中,采用NGS方法的实验室显示,在BRAF测试中实现正确结果的可能性要大得多,这再次受到更可靠地检测V600K变化的能力的推动[98]。最终,NGS检测具有更大的成本效益和更短的总体周转时间的证据[99],可以为跨肿瘤类型的更多潜在靶标提供信息,以及许多FDA批准的NGS检测的可用性[100],将可能继续推动该领域摆脱对单基因检测的依赖。
免疫组织化学(IHC)
使用VE1克隆的突变特异性BRAF V600E IHC是一种公认的黑色素瘤筛查工具。相对于分子检测,VE1 IHC的灵敏度范围为85%~97%,特异性范围为96%~100%,具体取决于定义的对照[101,102]。IHC检测提供的较短周转时间可以使患者比依赖分子检测时更早开始BRAF抑制剂治疗——这可以为疾病快速进展的患者提供临床获益。然而,鉴于较少通常建议进行确证性分子检测,以改善性能特征[58]。转移性病变和色素性肿瘤最常引起IHC——分子差异[103]。骨样本中转移性黑色素瘤的脱钙可能导致IHC和/或分子检测结果假阴性,并且可能是这些方法之间差异的来源[104,105]。VE1 IHC对BRAF V600K(和其他非V600E突变)也不敏感;因此,需要突变谱分析来检测这些改变[103]。
对于甲状腺癌,VE1 IHC已被验证可用于检测组织和细胞学细胞块标本中的BRAF V600E突变[103,106];然而,该抗体在直接涂片和液体制剂中的表现有限,特别是那些含有模糊元素的制剂[107,108]。VE1免疫组化染色的特异性在碰撞瘤中得到证实,其中包括一例BRAF-野生型肺腺癌和一例转移性BRAF V600E突变的乳头状甲状腺癌(图3)。
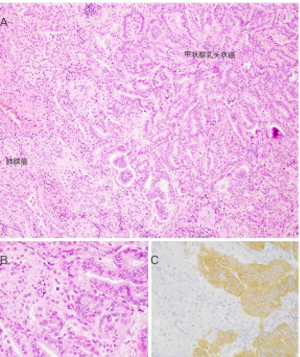
已经建议将BRAF IHC作为检测结肠锯齿状病变的工具[109]以及筛查BRAF V600E突变的结直肠癌[110]。对这种IHC抗体在结肠肿瘤队列中的早期研究得出了相互矛盾的结果,一个使用杂交瘤上清液[109]的实验室得出了非常有希望的结果,但有证据表明,使用商用抗体[111-113]对来自不同群体的BRAF突变的结肠癌的敏感性和特异性很差。其他小组观察到VE1、IHC和分子检测可以显示很高的一致性,但需要严格优化IHC检测,即便如此,仍有相当数量的弱染色病例需要通过分子方法进行确认[103,114]。分子分析,包括全面的下一代测序测试,可以同时提供各种潜在生物标记物的信息,它可能会取代BRAF IHC,因为它只提供一个与治疗相关的变量的信息。然而,理论上,BRAF VE1 IHC结合MMR蛋白评估可以为结肠腺癌患者的一线或后期治疗决策提供大多数必要的信息(参见上文的治疗意义)。
在非小细胞肺癌,特别是肺腺癌中检测BRAF V600E突变,现已成为联合应用BRAF/MEK抑制剂进行一线治疗的适应证。这一临床适应症重新激发了人们对使用VE1 IHC快速筛查/检测这种肿瘤类型的BRAF V600E突变的兴趣。
V600E以外的V600密码子突变在NSCLC中异常罕见;因此,影响黑色素瘤检测的临床假阴性问题与该肿瘤类型无关。鉴于BRAF V600E突变在NSCLC中的罕见性,早期研究中仅有少量真阳性病例[115,116],因此尽管性能特征相对稳健,但数据有限,且临床试验指南通常不鼓励这种检测方法[117]。最近多项使用较大分子定义队列的研究证实了VE1检测在NSCLC中的实用性,灵敏度范围为96.6%~100%,特异性范围为98.6%~100%。因此,可以预期VE1 IHC可以应用于常规的基于IHC的预测性生物标志物筛查[118,119]。
总结
综上所述,BRAF是MAPK信号通路的中心成员,MAPK信号通路的激活驱动促进生存和增殖的程序。重要的是,BRAF的单独激活似乎不足以驱动恶性肿瘤行为,相反,它使癌基因驱动的衰老程序得以实现,表现为缓慢的克隆过程,如良性痣或发生在不同身体部位的腺瘤。在BRAF激活事件的背景下,恶性转化似乎需要第二次打击;在该转化步骤中涉及的基因包括CDKN2A、PTEN和TP53。根据现有的功能证据,BRAF的基因组改变,包括众所周知的点突变(包括V600),以及小的Indels和更大的结构变异,根据其作为单体、二聚体或通过RAS依赖性触发下游信号的能力,被视为1、2或3类。这一分类对BRAF靶向治疗的疗效具有重要意义,BRAF靶向治疗似乎对那些携带1类突变的肿瘤最有效。
BRAF在各种基因组背景下基本上发生在每个身体部位的肿瘤中发生突变或其他改变,引起病理和临床肿瘤表现的巨大多样性。对于晚期疾病患者,RAF靶向治疗的疗效取决于发现BRAF突变的背景,最大的临床疗效来自针对RAF和其他上游(EGFR)或下游(MEK/ERK)信号的联合治疗。鉴于BRAF在人类肿瘤中的变化具有广泛的临床意义,准确和灵敏的生物标志物检测在实践中是必不可少的。已经开发并优化了一系列检测方法,从靶向PCR分子诊断到BRAF V600E突变特异性IHC再到高通量下一代测序。这些不同的方法都有其优缺点,但分子方法往往表现出非常相似的性能特征,无论其作为实验室检查或FDA标记的伴随诊断的状态如何。实验室可以选择使用多种检测方法来利用各自的优势,例如周转时间、灵敏度或广度。
正在进行的针对BRAF驱动的恶性肿瘤患者治疗的研究将可能继续侧重于优化靶向抑制剂的组合,并将至少在一部分BRAF驱动的肿瘤中利用免疫治疗。然而,我们目前对这些疗法的内在和获得性耐药性的理解还不成熟,在所有可能性分析中,将需要对超出基因组、进入甲基化组、蛋白质组和超越的肿瘤进行分析,以更充分地解析负责的通路。
Acknowledgments
Funding: None.
Footnote
Provenance and Peer Review: This article was commissioned by the Guest Editors Mari Mino-Kenudson and Yin (Rex) P. Hung for the series “Predictive and Prognostic Biomarkers in Tumors” published in Precision Cancer Medicine. The article has undergone external peer review.
Reporting Checklist: The author has completed the Narrative Review reporting checklist. Available at http://dx.doi.org/10.21037/pcm-20-39
Conflicts of Interest: The author has completed the ICMJE uniform disclosure form (available at http://dx.doi.org/10.21037/pcm-20-39). The series “Predictive and Prognostic Biomarkers in Tumors” was commissioned by the editorial office without any funding or sponsorship. LMS reports grants from Genentech, personal fees from EMD Serono, personal fees from Foghorn Therapeutics, and personal fees from AstraZeneca, outside the submitted work. The author has no other conflicts of interest to declare.
Ethical Statement: The author is accountable for all aspects for the work in ensuring that questions related to the accuracy or integrity of any part of the work are appropriately investigated and resolved.
Open Access Statement: This is an Open Access article distributed in accordance with the Creative Commons Attribution-NonCommercial-NoDerivs 4.0 International License (CC BY-NC-ND 4.0), which permits the non-commercial replication and distribution of the article with the strict proviso that no changes or edits are made and the original work is properly cited (including links to both the formal publication through the relevant DOI and the license). See: https://creativecommons.org/licenses/by-nc-nd/4.0/.
References
- Dankner M, Rose AAN, Rajkumar S, et al. Classifying BRAF alterations in cancer: new rational therapeutic strategies for actionable mutations. Oncogene 2018;37:3183-99. [Crossref] [PubMed]
- Marais R, Light Y, Paterson HF, et al. Differential regulation of Raf-1, A-Raf, and B-Raf by oncogenic ras and tyrosine kinases. J Biol Chem 1997;272:4378-83. [Crossref] [PubMed]
- Niemeyer CM. RAS diseases in children. Haematologica 2014;99:1653-62. [Crossref] [PubMed]
- Kratz CP, Franke L, Peters H, et al. Cancer spectrum and frequency among children with Noonan, Costello, and cardio-facio-cutaneous syndromes. Br J Cancer 2015;112:1392-7. [Crossref] [PubMed]
- Yao Z, Yaeger R, Rodrik-Outmezguine VS, et al. Tumours with class 3 BRAF mutants are sensitive to the inhibition of activated RAS. Nature 2017;548:234-8. [Crossref] [PubMed]
- Davies H, Bignell GR, Cox C, et al. Mutations of the BRAF gene in human cancer. Nature 2002;417:949-54. [Crossref] [PubMed]
- Pollock PM, Harper UL, Hansen KS, et al. High frequency of BRAF mutations in nevi. Nat Genet 2003;33:19-20. [Crossref] [PubMed]
- Michaloglou C, Vredeveld LC, Soengas MS, et al. BRAFE600-associated senescence-like cell cycle arrest of human naevi. Nature 2005;436:720-4. [Crossref] [PubMed]
- Damsky WE, Bosenberg M. Melanocytic nevi and melanoma: unraveling a complex relationship. Oncogene 2017;36:5771-92. [Crossref] [PubMed]
- Patel PL, Suram A, Mirani N, et al. Derepression of hTERT gene expression promotes escape from oncogene-induced cellular senescence. Proc Natl Acad Sci U S A 2016;113:E5024-33. [Crossref] [PubMed]
- Chui MH, Shih IM. Oncogenic BRAF and KRAS mutations in endosalpingiosis. J Pathol 2020;250:148-58. [Crossref] [PubMed]
- Chan E, Stohr BA, Croom NA, et al. Molecular characterisation of metanephric adenomas beyond BRAF: genetic evidence for potential malignant evolution. Histopathology 2020;76:1084-90. [Crossref] [PubMed]
- Martinez-Barbera JP, Andoniadou CL. Biological Behaviour of Craniopharyngiomas. Neuroendocrinology 2020;110:797-804. [Crossref] [PubMed]
- Koelsche C, Wohrer A, Jeibmann A, et al. Mutant BRAF V600E protein in ganglioglioma is predominantly expressed by neuronal tumor cells. Acta Neuropathol 2013;125:891-900. [Crossref] [PubMed]
- Chen J, Jian X, Deng S, et al. Identification of recurrent USP48 and BRAF mutations in Cushing's disease. Nat Commun 2018;9:3171. [Crossref] [PubMed]
- Chang JC, Montecalvo J, Borsu L, et al. Bronchiolar Adenoma: Expansion of the Concept of Ciliated Muconodular Papillary Tumors With Proposal for Revised Terminology Based on Morphologic, Immunophenotypic, and Genomic Analysis of 25 Cases. Am J Surg Pathol 2018;42:1010-26. [Crossref] [PubMed]
- Kim L, Kim YS, Lee JS, et al. Ciliated muconodular papillary tumor of the lung harboring BRAF V600E mutation and p16(INK4a) overexpression without proliferative activity may represent an example of oncogene-induced senescence. J Thorac Dis 2017;9:E1039-44. [Crossref] [PubMed]
- Cavalli G, Biavasco R, Borgiani B, et al. Oncogene-induced senescence as a new mechanism of disease: the paradigm of erdheim-chester disease. Front Immunol 2014;5:281. [Crossref] [PubMed]
- Roden AC, Hu X, Kip S, et al. BRAF V600E expression in Langerhans cell histiocytosis: clinical and immunohistochemical study on 25 pulmonary and 54 extrapulmonary cases. Am J Surg Pathol 2014;38:548-51. [Crossref] [PubMed]
- Kriegl L, Neumann J, Vieth M, et al. Up and downregulation of p16(Ink4a) expression in BRAF-mutated polyps/adenomas indicates a senescence barrier in the serrated route to colon cancer. Mod Pathol 2011;24:1015-22. [Crossref] [PubMed]
- Zeppernick F, Ardighieri L, Hannibal CG, et al. BRAF mutation is associated with a specific cell type with features suggestive of senescence in ovarian serous borderline (atypical proliferative) tumors. Am J Surg Pathol 2014;38:1603-11. [Crossref] [PubMed]
- Haroche J, Cohen-Aubart F, Amoura Z. Erdheim-Chester disease. Blood 2020;135:1311-8. [Crossref] [PubMed]
- Diamond EL, Durham BH, Haroche J, et al. Diverse and Targetable Kinase Alterations Drive Histiocytic Neoplasms. Cancer Discov 2016;6:154-65. [Crossref] [PubMed]
- Rodriguez-Galindo C, Allen CE. Langerhans cell histiocytosis. Blood 2020;135:1319-31. [Crossref] [PubMed]
- Chakraborty R, Hampton OA, Shen X, et al. Mutually exclusive recurrent somatic mutations in MAP2K1 and BRAF support a central role for ERK activation in LCH pathogenesis. Blood 2014;124:3007-15. [Crossref] [PubMed]
- McGinnis LM, Nybakken G, Ma L, et al. Frequency of MAP2K1, TP53, and U2AF1 Mutations in BRAF-mutated Langerhans Cell Histiocytosis: Further Characterizing the Genomic Landscape of LCH. Am J Surg Pathol 2018;42:885-90. [Crossref] [PubMed]
- Chilosi M, Facchetti F, Calio A, et al. Oncogene-induced senescence distinguishes indolent from aggressive forms of pulmonary and non-pulmonary Langerhans cell histiocytosis. Leuk Lymphoma 2014;55:2620-6. [Crossref] [PubMed]
- O'Brien MJ, Yang S, Mack C, et al. Comparison of microsatellite instability, CpG island methylation phenotype, BRAF and KRAS status in serrated polyps and traditional adenomas indicates separate pathways to distinct colorectal carcinoma end points. Am J Surg Pathol 2006;30:1491-501. [Crossref] [PubMed]
- Murakami T, Mitomi H, Saito T, et al. Distinct WNT/beta-catenin signaling activation in the serrated neoplasia pathway and the adenoma-carcinoma sequence of the colorectum. Mod Pathol 2015;28:146-58. [Crossref] [PubMed]
- Yozu M, Kem M, Cenaj O, et al. Loss of expression of MLH1 in non-dysplastic crypts is a harbinger of neoplastic progression in sessile serrated adenomas/polyps. Histopathology 2019;75:376-84. [Crossref] [PubMed]
- Murakami T, Akazawa Y, Yatagai N, et al. Molecular characterization of sessile serrated adenoma/polyps with dysplasia/carcinoma based on immunohistochemistry, next-generation sequencing, and microsatellite instability testing: a case series study. Diagn Pathol 2018;13:88. [Crossref] [PubMed]
- Cerami E, Gao J, Dogrusoz U, et al. The cBio cancer genomics portal: an open platform for exploring multidimensional cancer genomics data. Cancer Discov 2012;2:401-4. [Crossref] [PubMed]
- Maraka S, Janku F. BRAF alterations in primary brain tumors. Discov Med 2018;26:51-60. [PubMed]
- Vogelstein B, Papadopoulos N, Velculescu VE, et al. Cancer genome landscapes. Science 2013;339:1546-58. [Crossref] [PubMed]
- Dagogo-Jack I, Martinez P, Yeap BY, et al. Impact of BRAF Mutation Class on Disease Characteristics and Clinical Outcomes in BRAF-mutant Lung Cancer. Clin Cancer Res 2019;25:158-65. [Crossref] [PubMed]
- Dankner M, Lajoie M, Moldoveanu D, et al. Dual MAPK Inhibition Is an Effective Therapeutic Strategy for a Subset of Class II BRAF Mutant Melanomas. Clin Cancer Res 2018;24:6483-94. [Crossref] [PubMed]
- Ciampi R, Knauf JA, Kerler R, et al. Oncogenic AKAP9-BRAF fusion is a novel mechanism of MAPK pathway activation in thyroid cancer. J Clin Invest 2005;115:94-101. [Crossref] [PubMed]
- Jones DT, Kocialkowski S, Liu L, et al. Tandem duplication producing a novel oncogenic BRAF fusion gene defines the majority of pilocytic astrocytomas. Cancer Res 2008;68:8673-7. [Crossref] [PubMed]
- Cin H, Meyer C, Herr R, et al. Oncogenic FAM131B-BRAF fusion resulting from 7q34 deletion comprises an alternative mechanism of MAPK pathway activation in pilocytic astrocytoma. Acta Neuropathol 2011;121:763-74. [Crossref] [PubMed]
- Chmielecki J, Hutchinson KE, Frampton GM, et al. Comprehensive genomic profiling of pancreatic acinar cell carcinomas identifies recurrent RAF fusions and frequent inactivation of DNA repair genes. Cancer Discov 2014;4:1398-405. [Crossref] [PubMed]
- Ross JS, Wang K, Chmielecki J, et al. The distribution of BRAF gene fusions in solid tumors and response to targeted therapy. Int J Cancer 2016;138:881-90. [Crossref] [PubMed]
- Archer Quiver Fusion Database [updated May 1, 2018]. Available online: http://quiver.archerdx.com/results?query=braf.
- Kemper K, Krijgsman O, Kong X, et al. BRAF(V600E) Kinase Domain Duplication Identified in Therapy-Refractory Melanoma Patient-Derived Xenografts. Cell Rep 2016;16:263-77. [Crossref] [PubMed]
- Wegert J, Vokuhl C, Collord G, et al. Recurrent intragenic rearrangements of EGFR and BRAF in soft tissue tumors of infants. Nat Commun 2018;9:2378. [Crossref] [PubMed]
- Sievert AJ, Lang SS, Boucher KL, et al. Paradoxical activation and RAF inhibitor resistance of BRAF protein kinase fusions characterizing pediatric astrocytomas. Proc Natl Acad Sci U S A 2013;110:5957-62. [Crossref] [PubMed]
- Yao Z, Gao Y, Su W, et al. RAF inhibitor PLX8394 selectively disrupts BRAF dimers and RAS-independent BRAF-mutant-driven signaling. Nat Med 2019;25:284-91. [Crossref] [PubMed]
- Curtin JA, Fridlyand J, Kageshita T, et al. Distinct sets of genetic alterations in melanoma. N Engl J Med 2005;353:2135-47. [Crossref] [PubMed]
- Cancer Genome Atlas Network. Genomic Classification of Cutaneous Melanoma. Cell 2015;161:1681-96. [Crossref] [PubMed]
- Flaherty KT, Puzanov I, Kim KB, et al. Inhibition of mutated, activated BRAF in metastatic melanoma. N Engl J Med 2010;363:809-19. [Crossref] [PubMed]
- Chapman PB, Hauschild A, Robert C, et al. Improved survival with vemurafenib in melanoma with BRAF V600E mutation. N Engl J Med 2011;364:2507-16. [Crossref] [PubMed]
- Sosman JA, Kim KB, Schuchter L, et al. Survival in BRAF V600-mutant advanced melanoma treated with vemurafenib. N Engl J Med 2012;366:707-14. [Crossref] [PubMed]
- McArthur GA, Chapman PB, Robert C, et al. Safety and efficacy of vemurafenib in BRAF(V600E) and BRAF(V600K) mutation-positive melanoma (BRIM-3): extended follow-up of a phase 3, randomised, open-label study. Lancet Oncol 2014;15:323-32. [Crossref] [PubMed]
- Nazarian R, Shi H, Wang Q, et al. Melanomas acquire resistance to B-RAF(V600E) inhibition by RTK or N-RAS upregulation. Nature 2010;468:973-7. [Crossref] [PubMed]
- Sun C, Wang L, Huang S, et al. Reversible and adaptive resistance to BRAF(V600E) inhibition in melanoma. Nature 2014;508:118-22. [Crossref] [PubMed]
- Shi H, Hugo W, Kong X, et al. Acquired resistance and clonal evolution in melanoma during BRAF inhibitor therapy. Cancer Discov 2014;4:80-93. [Crossref] [PubMed]
- Robert C, Karaszewska B, Schachter J, et al. Improved overall survival in melanoma with combined dabrafenib and trametinib. N Engl J Med 2015;372:30-9. [Crossref] [PubMed]
- Johnson DB, Flaherty KT, Weber JS, et al. Combined BRAF (Dabrafenib) and MEK inhibition (Trametinib) in patients with BRAFV600-mutant melanoma experiencing progression with single-agent BRAF inhibitor. J Clin Oncol 2014;32:3697-704. [Crossref] [PubMed]
- Cutaneous Melanoma 2020 [updated May 18, 2020; cited 2020]. Version 3.2020. Available online: https://www.nccn.org/professionals/physician_gls/pdf/cutaneous_melanoma.pdf
- Puzanov I, Ribas A, Robert C, et al. Association of BRAF V600E/K Mutation Status and Prior BRAF/MEK Inhibition With Pembrolizumab Outcomes in Advanced Melanoma: Pooled Analysis of 3 Clinical Trials. JAMA Oncol 2020;6:1256-64. [Crossref] [PubMed]
- Hyman DM, Puzanov I, Subbiah V, et al. Vemurafenib in Multiple Nonmelanoma Cancers with BRAF V600 Mutations. N Engl J Med 2015;373:726-36. [Crossref] [PubMed]
- De Roock W, Claes B, Bernasconi D, et al. Effects of KRAS, BRAF, NRAS, and PIK3CA mutations on the efficacy of cetuximab plus chemotherapy in chemotherapy-refractory metastatic colorectal cancer: a retrospective consortium analysis. Lancet Oncol 2010;11:753-62. [Crossref] [PubMed]
- Shinozaki E, Yoshino T, Yamazaki K, et al. Clinical significance of BRAF non-V600E mutations on the therapeutic effects of anti-EGFR monoclonal antibody treatment in patients with pretreated metastatic colorectal cancer: the Biomarker Research for anti-EGFR monoclonal Antibodies by Comprehensive Cancer genomics (BREAC) study. Br J Cancer 2017;117:1450-8. [Crossref] [PubMed]
- Prahallad A, Sun C, Huang S, et al. Unresponsiveness of colon cancer to BRAF(V600E) inhibition through feedback activation of EGFR. Nature 2012;483:100-3. [Crossref] [PubMed]
- Corcoran RB, Ebi H, Turke AB, et al. EGFR-mediated re-activation of MAPK signaling contributes to insensitivity of BRAF mutant colorectal cancers to RAF inhibition with vemurafenib. Cancer Discov 2012;2:227-35. [Crossref] [PubMed]
- Corcoran RB, Atreya CE, Falchook GS, et al. Combined BRAF and MEK Inhibition With Dabrafenib and Trametinib in BRAF V600-Mutant Colorectal Cancer. J Clin Oncol 2015;33:4023-31. [Crossref] [PubMed]
- Corcoran RB, Andre T, Atreya CE, et al. Combined BRAF, EGFR, and MEK Inhibition in Patients with BRAF(V600E)-Mutant Colorectal Cancer. Cancer Discov 2018;8:428-43. [Crossref] [PubMed]
- Kopetz S, Grothey A, Yaeger R, et al. Encorafenib, Binimetinib, and Cetuximab in BRAF V600E-Mutated Colorectal Cancer. N Engl J Med 2019;381:1632-43. [Crossref] [PubMed]
- Huijberts SC, van Geel RM, Bernards R, et al. Encorafenib, binimetinib and cetuximab combined therapy for patients with BRAFV600E mutant metastatic colorectal cancer. Future Oncol 2020;16:161-73. [Crossref] [PubMed]
- FDA approves encorafenib in combination with cetuximab for metastatic colorectal cancer with a BRAF V600E mutation 2020 [updated 4/9/2020]. Available online: https://www.fda.gov/drugs/resources-information-approved-drugs/fda-approves-encorafenib-combination-cetuximab-metastatic-colorectal-cancer-braf-v600e-mutation
- Shinozaki E, Yoshino T, Tsuchihara K. Reply to `Comment on `Clinical significance of BRAF non-V600E mutations on the therapeutic effects of anti-EGFR monoclonal antibody treatment in patients with pretreated metastatic colorectal cancer: the Biomarker Research for anti-EGFR monoclonal Antibodies by Comprehensive Cancer genomics (BREAC) study''. Br J Cancer 2018;118:1278-9. [Crossref] [PubMed]
- Jones JC, Renfro LA, Al-Shamsi HO, et al. (Non-V600) BRAF Mutations Define a Clinically Distinct Molecular Subtype of Metastatic Colorectal Cancer. J Clin Oncol 2017;35:2624-30. [Crossref] [PubMed]
- Dankner M, Rose AAN. Comment on 'Clinical significance of BRAF non-V600E mutations on the therapeutic effects of anti-EGFR monoclonal antibody treatment in patients with pretreated metastatic colorectal cancer: the Biomarker Research for anti-EGFR monoclonal Antibodies by Comprehensive Cancer genomics (BREAC) study'. Br J Cancer 2018;118:1276-7. [Crossref] [PubMed]
- Parsons MT, Buchanan DD, Thompson B, et al. Correlation of tumour BRAF mutations and MLH1 methylation with germline mismatch repair (MMR) gene mutation status: a literature review assessing utility of tumour features for MMR variant classification. J Med Genet 2012;49:151-7. [Crossref] [PubMed]
- Lochhead P, Kuchiba A, Imamura Y, et al. Microsatellite instability and BRAF mutation testing in colorectal cancer prognostication. J Natl Cancer Inst 2013;105:1151-6. [Crossref] [PubMed]
- Phipps AI, Alwers E, Harrison T, et al. Association Between Molecular Subtypes of Colorectal Tumors and Patient Survival, Based on Pooled Analysis of 7 International Studies. Gastroenterology 2020;158:2158-68.e4. [Crossref] [PubMed]
- Le DT, Uram JN, Wang H, et al. PD-1 Blockade in Tumors with Mismatch-Repair Deficiency. N Engl J Med 2015;372:2509-20. [Crossref] [PubMed]
- Le DT, Kim TW, Van Cutsem E, et al. Phase II Open-Label Study of Pembrolizumab in Treatment-Refractory, Microsatellite Instability-High/Mismatch Repair-Deficient Metastatic Colorectal Cancer: KEYNOTE-164. J Clin Oncol 2020;38:11-9. [Crossref] [PubMed]
- Andre T, Shiu KK, Kim TW, et al. Pembrolizumab versus chemotherapy for microsatellite instability-high/mismatch repair deficient metastatic colorectal cancer: The phase 3 KEYNOTE-177 Study. J Clin Oncol 2020;38:LBA4. [Crossref]
- FDA approves pembrolizumab for first-line treatment of MSI-H/dMMR colorectal cancer 2020 [updated 6/30/2020]. Available online: https://www.fda.gov/drugs/drug-approvals-and-databases/fda-approves-pembrolizumab-first-line-treatment-msi-hdmmr-colorectal-cancer
- Odogwu L, Mathieu L, Blumenthal G, et al. FDA Approval Summary: Dabrafenib and Trametinib for the Treatment of Metastatic Non-Small Cell Lung Cancers Harboring BRAF V600E Mutations. Oncologist 2018;23:740-5. [Crossref] [PubMed]
- Planchard D, Smit EF, Groen HJM, et al. Dabrafenib plus trametinib in patients with previously untreated BRAF(V600E)-mutant metastatic non-small-cell lung cancer: an open-label, phase 2 trial. Lancet Oncol 2017;18:1307-16. [Crossref] [PubMed]
- Planchard D, Besse B, Groen H, et al. Updated overall survival (OS) and genomic analysis from a single-arm phase II study of dabrafenib (D) + trametinib (T) in patients (pts) with BRAF V600E mutant (Mut) metastatic non-small cell lung cancer (NSCLC). J Clin Oncol 2020;38:9593. [Crossref]
- Dudnik E, Peled N, Nechushtan H, et al. BRAF Mutant Lung Cancer: Programmed Death Ligand 1 Expression, Tumor Mutational Burden, Microsatellite Instability Status, and Response to Immune Check-Point Inhibitors. J Thorac Oncol 2018;13:1128-37. [Crossref] [PubMed]
- Dagogo-Jack I, Awad MM, Shaw AT. BRAF Mutation Class and Clinical Outcomes-Response. Clin Cancer Res 2019;25:3189. [Crossref] [PubMed]
- FDA approves new uses for two drugs administered together for the treatment of BRAF-positive anaplastic thyroid cancer 2018 [updated 5/4/2018]. Available online: https://www.fda.gov/news-events/press-announcements/fda-approves-new-uses-two-drugs-administered-together-treatment-braf-positive-anaplastic-thyroid
- Subbiah V, Kreitman RJ, Wainberg ZA, et al. Dabrafenib and Trametinib Treatment in Patients With Locally Advanced or Metastatic BRAF V600-Mutant Anaplastic Thyroid Cancer. J Clin Oncol 2018;36:7-13. [Crossref] [PubMed]
- Diamond EL, Subbiah V, Lockhart AC, et al. Vemurafenib for BRAF V600-Mutant Erdheim-Chester Disease and Langerhans Cell Histiocytosis: Analysis of Data From the Histology-Independent, Phase 2, Open-label VE-BASKET Study. JAMA Oncol 2018;4:384-8. [Crossref] [PubMed]
- Oneal PA, Kwitkowski V, Luo L, et al. FDA Approval Summary: Vemurafenib for the Treatment of Patients with Erdheim-Chester Disease with the BRAFV600 Mutation. Oncologist 2018;23:1520-4. [Crossref] [PubMed]
- Brunet M, Khalifa E, Italiano A. Enabling Precision Medicine for Rare Head and Neck Tumors: The Example of BRAF/MEK Targeting in Patients With Metastatic Ameloblastoma. Front Oncol 2019;9:1204. [Crossref] [PubMed]
- Cuviello A, Goyal A, Zick A, et al. Sporadic Malignant Glomus Tumor of the Brachial Plexus With Response to Targeted Therapy Directed Against Oncogenic BRAF. JCO Precis Oncol. 2018;2018.
- Groisberg R, Hong DS, Roszik J, et al. Clinical Next-Generation Sequencing for Precision Oncology in Rare Cancers. Mol Cancer Ther 2018;17:1595-601. [Crossref] [PubMed]
- Roche Cobas DNA Sample Preparation Kit, COBAS 4800 BRAF V600 mutation test 2020 [updated 7/27/2020]. Available online: https://www.accessdata.fda.gov/scripts/cdrh/cfdocs/cfpma/pma.cfm?id=P110020S016
- Qu K, Pan Q, Zhang X, et al. Detection of BRAF V600 mutations in metastatic melanoma: comparison of the Cobas 4800 and Sanger sequencing assays. J Mol Diagn 2013;15:790-5. [Crossref] [PubMed]
- Lopez-Rios F, Angulo B, Gomez B, et al. Comparison of testing methods for the detection of BRAF V600E mutations in malignant melanoma: pre-approval validation study of the companion diagnostic test for vemurafenib. PLoS One 2013;8:e53733. [Crossref] [PubMed]
- Anderson S, Bloom KJ, Vallera DU, et al. Multisite analytic performance studies of a real-time polymerase chain reaction assay for the detection of BRAF V600E mutations in formalin-fixed, paraffin-embedded tissue specimens of malignant melanoma. Arch Pathol Lab Med 2012;136:1385-91. [Crossref] [PubMed]
- Therascreen BRAF V600E RGQ PCR Kit 2020 [updated 7/27/2020]. Available online: https://www.accessdata.fda.gov/scripts/cdrh/cfdocs/cfpma/pma.cfm?id=P190026
- Moncur JT, Bartley AN, Bridge JA, et al. Performance Comparison of Different Analytic Methods in Proficiency Testing for Mutations in the BRAF, EGFR, and KRAS Genes: A Study of the College of American Pathologists Molecular Oncology Committee. Arch Pathol Lab Med 2019;143:1203-11. [Crossref] [PubMed]
- Surrey LF, Oakley FD, Merker JD, et al. Next-Generation Sequencing (NGS) Methods Show Superior or Equivalent Performance to Non-NGS Methods on BRAF, EGFR, and KRAS Proficiency Testing Samples. Arch Pathol Lab Med 2019;143:980-4. [Crossref] [PubMed]
- Pennell NA, Mutebi A, Zhou ZY, et al. Economic Impact of Next-Generation Sequencing Versus Single-Gene Testing to Detect Genomic Alterations in Metastatic Non–Small-Cell Lung Cancer Using a Decision Analytic Model. JCO Precision Oncology 2019;3:1-9. [Crossref]
- Nucleic Acid Based Tests 2020 [updated 7/7/2020]. Available online: https://www.fda.gov/medical-devices/vitro-diagnostics/nucleic-acid-based-tests
- Pearlstein MV, Zedek DC, Ollila DW, et al. Validation of the VE1 immunostain for the BRAF V600E mutation in melanoma. J Cutan Pathol 2014;41:724-32. [Crossref] [PubMed]
- Schirosi L, Strippoli S, Gaudio F, et al. Is immunohistochemistry of BRAF V600E useful as a screening tool and during progression disease of melanoma patients? BMC Cancer 2016;16:905. [Crossref] [PubMed]
- Fisher KE, Cohen C, Siddiqui MT, et al. Accurate detection of BRAF p.V600E mutations in challenging melanoma specimens requires stringent immunohistochemistry scoring criteria or sensitive molecular assays. Hum Pathol 2014;45:2281-93. [Crossref] [PubMed]
- Rapisuwon S, Busam KJ, Parks K, Chapman PB, Lee E, Atkins MB. Discordance Between Cobas BRAF V600 Testing and VE1 Immunohistochemistry in a Melanoma Patient With Bone Marrow Metastases. Am J Dermatopathol 2016;38:687-9. [Crossref] [PubMed]
- Bourhis A, Le Flahec G, Uguen A. Decalcification can cause the failure of BRAF molecular analyses and anti-BRAFV600E VE1 immunohistochemistry. Pathol Int 2019;69:219-23. [Crossref] [PubMed]
- Zimmermann AK, Camenisch U, Rechsteiner MP, et al. Value of immunohistochemistry in the detection of BRAF(V600E) mutations in fine-needle aspiration biopsies of papillary thyroid carcinoma. Cancer Cytopathol 2014;122:48-58. [Crossref] [PubMed]
- Wobker SE, Kim LT, Hackman TG, et al. Use of BRAF v600e immunocytochemistry on FNA direct smears of papillary thyroid carcinoma. Cancer Cytopathol 2015;123:531-9. [Crossref] [PubMed]
- Straccia P, Brunelli C, Rossi ED, et al. The immunocytochemical expression of VE-1 (BRAF V600E-related) antibody identifies the aggressive variants of papillary thyroid carcinoma on liquid-based cytology. Cytopathology 2019;30:460-7. [Crossref] [PubMed]
- Mesteri I, Bayer G, Meyer J, et al. Improved molecular classification of serrated lesions of the colon by immunohistochemical detection of BRAF V600E. Mod Pathol 2014;27:135-44. [Crossref] [PubMed]
- Kwon JH, Jeong BK, Yoon YS, et al. Utility of BRAF VE1 Immunohistochemistry as a Screening Tool for Colorectal Cancer Harboring BRAF V600E Mutation. J Pathol Transl Med 2018;52:157-63. [Crossref] [PubMed]
- Adackapara CA, Sholl LM, Barletta JA, et al. Immunohistochemistry using the BRAF V600E mutation-specific monoclonal antibody VE1 is not a useful surrogate for genotyping in colorectal adenocarcinoma. Histopathology 2013;63:187-93. [Crossref] [PubMed]
- Panarelli NC, Weidner AS, Yantiss RK, et al. A cautionary note on the immunohistochemical detection of BRAF v600e mutations in serrated lesions of the colon. Mod Pathol 2015;28:740-1. [Crossref] [PubMed]
- Estrella JS, Tetzlaff MT, Bassett RL Jr, et al. Assessment of BRAF V600E Status in Colorectal Carcinoma: Tissue-Specific Discordances between Immunohistochemistry and Sequencing. Mol Cancer Ther 2015;14:2887-95. [Crossref] [PubMed]
- Bledsoe JR, Kamionek M, Mino-Kenudson M. BRAF V600E immunohistochemistry is reliable in primary and metastatic colorectal carcinoma regardless of treatment status and shows high intratumoral homogeneity. Am J Surg Pathol 2014;38:1418-28. [Crossref] [PubMed]
- Ilie M, Long E, Hofman V, et al. Diagnostic value of immunohistochemistry for the detection of the BRAFV600E mutation in primary lung adenocarcinoma Caucasian patients. Ann Oncol 2013;24:742-8. [Crossref] [PubMed]
- Sasaki H, Shimizu S, Tani Y, et al. Usefulness of immunohistochemistry for the detection of the BRAF V600E mutation in Japanese lung adenocarcinoma. Lung Cancer 2013;82:51-4. [Crossref] [PubMed]
- Lindeman NI, Cagle PT, Aisner DL, et al. Updated Molecular Testing Guideline for the Selection of Lung Cancer Patients for Treatment With Targeted Tyrosine Kinase Inhibitors: Guideline From the College of American Pathologists, the International Association for the Study of Lung Cancer, and the Association for Molecular Pathology. J Mol Diagn 2018;20:129-59. [Crossref] [PubMed]
- Gow CH, Hsieh MS, Lin YT, et al. Validation of Immunohistochemistry for the Detection of BRAF V600E-Mutated Lung Adenocarcinomas. Cancers (Basel) 2019;11:866. [Crossref] [PubMed]
- Hofman V, Benzaquen J, Heeke S, et al. Real-world assessment of the BRAF status in non-squamous cell lung carcinoma using VE1 immunohistochemistry: A single laboratory experience (LPCE, Nice, France). Lung Cancer 2020;145:58-62. [Crossref] [PubMed]
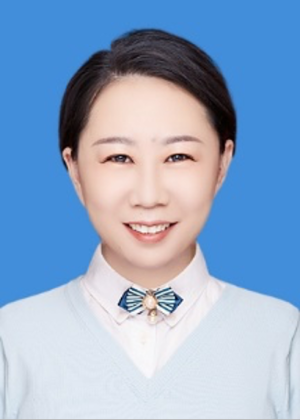
王琼
中国人民解放军总医院第一医学中心病理科,副主任技师,病理与病理生理学博士,兼任中华生物医学工程杂志、中华病理学杂志等审稿人。参与省部级以上课题3项,获实用新型专利3项,发表多篇SCI、中文文章。(更新时间:2021/9/5)
(本译文仅供学术交流,实际内容请以英文原文为准。)
Cite this article as: Sholl LM. A narrative review of BRAF alterations in human tumors: diagnostic and predictive implications. Precis Cancer Med 2020;3:26.