ALK disease: best first or later, and do we care about variants?
ALK+ disease: status quo and current challenges
ALK+ tumors are unique among metastatic non-small cell lung cancers (NSCLC) in many aspects: they have the lowest genetic complexity, with a mean tumor mutational burden (TMB) below 3 mutations/Mbp (1,2), require the most complex management at present, i.e., high-level expertise and close cooperation between medical oncology, interventional pneumology and radiology, thoracic surgery, radiation oncology as well as, molecular pathology over several years, and enjoy the best outcome. Even ALK+ NSCLC patients that received just one tyrosine-kinase inhibitor (TKI) have a better prognosis than their TKI-treated EGFR+ counterparts, while sequential ALK TKI administration meanwhile confers a median overall survival (OS) over 5 years, which is certainly one of the greatest successes in modern thoracic oncology (1,3). Instrumental for this remarkable achievement has been exquisitely rapid drug development, with already 5 routinely available ALK inhibitors spanning over 3 generations: crizotinib, ceritinib, alectinib, brigatinib, and lorlatinib (4). Outside the setting of clinical trials, these are used in variable sequences within the rapidly evolving frame of regulatory approval, and complemented with local-ablative treatments in case of oligoprogression (5). In this highly dynamic field, two major issues emerge currently as having key importance for further advances: the optimal use of already available treatment options, especially TKI, and the molecular characterization of early treatment failure in order to guide novel therapeutic approaches.
Best first or later?
A “good” drug generally combines efficacy with tolerability and safety (Figure 1A). Efficacy, in turn, is best assessed based on the progression-free survival (PFS) of first-line treatment, which is a composite measure of response rate and response duration in the clear setting of treatment-naivety. Thus, among several different compounds, “best” would be the drug with the longest first-line PFS (Figure 1A). Should this “best drug” lose efficacy when administered in the second line, it would be better to use it first (“best first”, Figure 1B), but if it would retain efficacy, it might be preferable to keep it for later (“best later”, Figure 1B). In the special scenario of our “best drug” causing non-druggable resistance, we might even choose to offer it as the last TKI line, after all other targeted therapy options have been exhausted (Figure 1B).
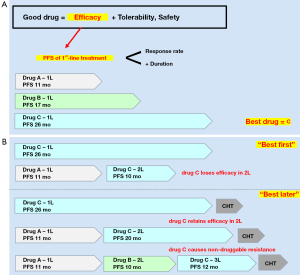
So, what is “best” for ALK+ disease today? Clearly, the two second-generation ALK inhibitors alectinib and brigatinib, which in randomized head-to-head comparisons vs. the first-generation compound crizotinib have demonstrated impressive superiority in first-line systemic efficacy, with similar objective response rates (ORR) about 80% and similar PFS hazard ratios (HR) of 0.5, suggesting similar median PFS intervals over 2 years (Table 1) (8,9,11,21). Of note, the ALEX trial, which has a longer follow-up, shows that the alectinib PFS curve flattens at about 50%, which makes the median first-line PFS relatively “unstable” (9,10) and highlights the superiority of PFS HR as efficacy measure in this context. It should also be noted here, that despite the overall superiority of alectinib, during the first 6 months the PFS curves of both arms in the ALEX trial run similarly (9). In contrast, other next-generation ALK TKI have either shown a shorter first-line PFS, like 17 months for ceritinib in the ASCEND-4 trial (7), or have single-arm, phase 2 data only, like ensartinib with 26 months (12), and lorlatinib with a promising first-line ORR of 90%, but still immature PFS data (Table 1) (13). The first-line brain efficacy of alectinib and brigatinib relative to crizotinib is even better, with PFS HRs of 0.4 and 0.27, respectively, and intracranial progression rates below 10% per year (Table 2) (9,11). Due to good central nervous system (CNS) penetration, brain ORR for all next-generation ALK TKI is actually very high, around 80%, similar to their systemic ORR (Table 1) and to brain ORR under radiotherapy, and much higher than the brain ORR of crizotinib or chemotherapy (Table 2) (6,22-26,29). This high brain efficacy of novel ALK inhibitors is especially important, because brain metastases affect a considerable fraction of ALK+ NSCLC patients, about 25% at initial diagnosis and 60% at 3 years if initially treated with crizotinib (30,31), and because these patients are younger, have a longer life expectancy and would suffer more from impairment of cognition, driving restrictions and reduced quality-of-life due to progressive CNS involvement.
Table 1
ALK TKI | Crizotinib | Ceritinib | Alectinib | Brigatinib | Ensartinib | Lorlatinib | |||
---|---|---|---|---|---|---|---|---|---|
First line | |||||||||
Study | PROFILE 1014 (6) | ASCEND-4 (7) | J-ALEX (8) | ALEX (9,10) | ALTA-1L (11) | FIH phase I/II (12) | Global phase II (13) (EXP-1) | ||
Comparator | Chemo | Chemo | Crizotinib | Crizotinib | Crizotinib | Single arm | Single arm | ||
Patients (N) | 172 | 189 | 103 | 152 | 137 | 15 | 30 | ||
ORR (%) | 74 | 73 | 76 | 83 | 76 | 80 | 90 | ||
Median PFS (months) | 10.9 | 16.6 | NR | 25.7* (34.8**) | NR | 26.2 | NR | ||
Hazard ratio | 0.45 | 0.50 | 0.34 | 0.50 | 0.49 | N/A | N/A | ||
Post-crizotinib | |||||||||
Study | ASCEND-1 (14) | ASCEND-2 (15) | Global phase II (16) | Phase II (17) | Phase I/II (18) | ALTA 90/180 (19,20) | FIH phase I/II (12) | Global phase II (13) (EXP-2/3A) | |
Patients (N) | 163 | 140 | 138 | 87 | 70 | 110 | 29 | 59 | |
ORR (%) | 56 | 38 | 50 | 48 | 71 | 55 | 69 | 70 | |
Median PFS (months) | 6.9 | 5.7 | 8.9 | 8.1 | 13.4 | 12.9/16.7 | 9 | NR | |
First-line PFS (months) | 26 | NR (probably ≥26)*** | 26 | NR | |||||
ΔPFS (2L-1L) (% of 1L) | −10 (60%) | −17 (65%) | At least −13 (at least 50%)*** | −17 (65%) | NA |
*, IRC-assessed 2017; **, INV-assessed 2018; ***, based on the similar PFS HR of alectinib and brigatinib in the ALEX and ALTA-1L trials against crizotinib. HR, hazard ratio; PFS, progression-free survival; 1L, first line; 2L, second line; FIH, first-in-human; NR, not reached; N/A, not applicable.
Table 2
ALK TKI or other treatment | Crizotinib | Ceritinib | Alectinib | Brigatinib | Ensartinib | Lorlatinib | Gefitinib/erlotinib | Osimertinib | Chemotherapy | Radiotherapy/+ TKI |
---|---|---|---|---|---|---|---|---|---|---|
First line | ||||||||||
Study | ALTA-1L (11), ALEX (9) | ASCEND-4 (7) (stable BM) | ALEX (9) (asympt. BM) | ALTA-1L (11) (asympt. BM) | FIH Ph. I/II (12) (asympt. BM) | global Ph. II (13) (EXP-1) | FLAURA (22) (stable BM) | ASCEND4 (7)-AURA3 (23)* | Various studies e.g., (24-26) | |
Patients (N) | 21–22 | 22 | 21 | 18 | 3 | 3 | 22 | |||
iORR (%) | 29–50 | 73 | 81 | 78 | 100 | 67 | 68 | 91 | 27–30 | ca. 50–60/85 |
Patients (N) | 138–151 | 152 | 137 | 277 | 279 | |||||
Intracranial progression at 1 year (%) | 19–41 | n/a | 9.4 | 8.8 | n/a | n/a | 24 | 8 | n/a | n/a |
Post-crizotinib | ||||||||||
Study | ASCEND-2 (15) | Ph. I/II studies (pooled) (27) | ALTA (180 mg) (28) | FIH Ph. I/II (12) | global Ph. II (13) (EXP2/3A) | AURA3 (23) | ||||
Patients (N) | 20 | 50 | 18 | 6 | 37 | 46 | ||||
iORR (%) | 45 | 64 | 67 | 83 | 68 | 70 | ||||
iORR (%) 1L | 74 | 81 | 78 | 100 | 75 | 91 | ||||
ΔORR (2L-1L), % (% of 1L) | −29 (39%) | −17 (21%) | −11 (14%) | −17 (17%) | −7 (9%) | −21 (23%) |
*, AURA3 patients were TKI-pretreated, but the control arm received platinum-based doublet chemotherapy, i.e., the typical first-line chemotherapy regimen. BM, brain metastases; ORR, objective response rate; iORR, intracranial ORR; ÄORR, difference in ORR; 1L, first-line; Ph., phase; FIH, first-in-human; n/a, not available; ca., circa.
On the other hand, if next-generation ALK inhibitors are given after crizotinib, they lose >50% of their systemic efficacy, with a PFS drop of 17 months for alectinib (16,17,32) and (estimated) at least 13 months of brigatinib (18-20,33), which is longer than the entire first-line PFS with crizotinib (Table 1). Second-line brain efficacy is also reduced, with a brain ORR for alectinib and brigatinib about 65% (Table 2) (15,27,28,34), considerably lower than the 80% noted in the first line (Table 1). More important, an additional about 20% of patients will experience brain progression under treatment with first-line crizotinib before they even get the opportunity to receive second-generation compounds, based of a brain progression rate of >20% per year with crizotinib and <10% per year with alectinib and brigatinib (Table 2). Thus, two main compelling arguments for a “best-first” approach with alectinib or brigatinib in ALK+ disease today are: (I) the longer median PFS with first-line alectinib or brigatinib compared to the composite PFS under first-line crizotinib followed by second-line alectinib or brigatinib (Table 1 and Figure 2); and (II) the greater delay of brain progression with upfront alectinib or brigatinib, with a PFS HR <0.5 compared to crizotinib, or <10% vs. >20% per year in absolute terms, respectively (Table 2). Consequently, and pending regulatory approval for upfront brigatinib, both the NCCN and ESMO guidelines currently recognize alectinib as a better first-line treatment option than crizotinib (35,36).
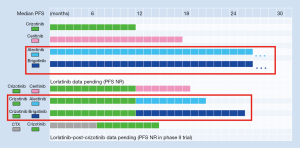
Besides, there are some additional, less straightforward, but also important arguments in favour of the “best-first” approach. First, a comparison among trials of next-generation ALK inhibitors shows that “best first” might increase the rate of long-term responses. For example, in the ALEX trial, the PFS curves for alectinib and crizotinib run similar for the first 6 months, but show an increasing deviation afterwards (9,10). Moreover, in the 2014 analysis of the ASCEND-1 trial, the PFS curve of treatment-naive patients receiving ceritinib flattened at about 50%, while the PFS curve of the crizotinib-pretreated patients decreased continuously, even though the difference in the median PFS of the two curves was negligible (37). A similar difference could be seen in the 2016 update of the same trial (14), and is also evident upon comparison of the PFS curves between the first- and the second-line alectinib (9,17), as well as between the first- and the second-line brigatinib trials (11,19). In all cases, upfront administration of the second-generation ALK inhibitor results in the tail of the PFS curve flattening at a higher level than if the same substance is administered after crizotinib, and this difference is more pronounced than the difference of the median PFS among the curves. Interestingly, upfront administration of second-generation TKI in case of chronic myeloid leukemia, the model disease of precision medicine, facilitates achievement of deep molecular remissions and successful drug discontinuation in a higher percentage of cases than imatinib (38). Admittedly, it will definitely take much more than a TKI to cure NSCLC, even in case of the less malignant ALK+ disease (1), but, still, second-generation ALK inhibitors are a more promising partner for definitive local treatments in oligometastatic cases, and for experimental approaches to eradicate widespread residual disease in other patients.
Along the same lines, some data suggest that chemotherapy could also impair benefit if administered upstream of “best-first” TKI. In the first report of the PROFILE-1014 trial in 2014 (6), there was a considerable difference of about 20% in the long-term PFS (i.e., in the tail of the PFS curve, beyond 2 years) between patients receiving crizotinib and chemotherapy as the first systemic treatment. In contrast, no such difference was evident when crizotinib and chemotherapy were both given after first-line chemotherapy in another trial (39), despite very similar PFS HRs in the two studies (0.45 vs. 0.49). Importantly, the final results of PROFILE-1014 published in 2018 showed that the aforementioned difference of about 20% was also reflected in the 5-year OS of the two patient groups despite crossover, i.e., patients that began with crizotinib fared better in the long run than patients that began with chemotherapy and received crizotinib subsequently (40). Such an impairment of TKI benefit after exposure to chemotherapy is presumably due to the genotoxic effect of cytostatics (41), since the accumulation of genetic abnormalities is crucial for the development of TKI failure (42-44), and possibly even more deleterious in the case of ALK+ disease, which has a particularly low baseline TMB (1). Based on these considerations, previous chemotherapy is likely to also impair the benefit from other TKI, including the currently “best drugs” alectinib and brigatinib, for which no formal first-line testing against chemotherapy will ever be performed. Concrete implications for clinical practice are: (I) that the results of molecular testing, including ALK status, should generally be awaited instead of “blind” initiation of chemotherapy for metastatic NSCLC; (II) that available TKI treatment options should generally be exhausted before resort to cytostatics; and (III) that not-yet-approved ALK inhibitors should ideally be accessible within compassionate use programs before administration of palliative chemotherapy.
What about radiotherapy and the “best-first” approach? A retrospective study of EGFR+ NSCLC patients with brain involvement published in 2017 by Magnuson et al. argued strongly for cerebral irradiation in addition to first-line TKI, but viewed from today, it actually provides good evidence for the opposite (45). In this study, the rate of brain progression was slightly higher for patients treated with TKI and stereotactic (SRT) or whole-brain radiotherapy (WBRT) compared to TKI alone, which also translated to a significant OS benefit. However, the rate of brain progression in all three patient groups (i.e., treated with first-/second-generation EGFR inhibitors with or without SRT or WBRT) was >20% per year, comparable to that observed in the similarly treated control arm of the FLAURA trial, and much higher than the <10% per year observed with osimertinib in the experimental arm of FLAURA (22). In other words, cross-trial comparison suggests a much stronger protective effect of osimertinib against brain progression compared to radiotherapy, which should therefore be probably reserved as salvage treatment at the time of osimertinib failure (Table 2). Upfront alectinib and brigatinib also confer a similar very low annual rate of brain progression below 10% (Table 1) (9,11), which suggests that ALK+ patients receiving “best-first” treatment can presumably also enjoy a “radiation-free” first line, even if asymptomatic and/or stable brain metastases are present (Table 2). Interestingly, recent data suggest that this probably holds true for large (>1 cm) or symptomatic brain lesions as well: even though such cases had been excluded from TKI trials, their treatment with alectinib in the routine setting showed an efficacy comparable to that observed in clinical studies (46). One exception, however, remains, and this concerns patients with oligometastatic disease to the brain, for which consolidative radiotherapy should still be offered, similar to the standard practice in case of extracranial oligometastases (47). In other patients, initial surveillance using magnetic resonance imaging appears to be preferable, with SRT to be offered as salvage treatment at the time of brain progression, since efficacy of next-generation ALK inhibitors in the second line is considerably lower and similar to first-line efficacy of erlotinib/gefitinib (Table 2), for which complementation with SRT improved survival in the study by Magnuson et al. (45). It should also be noted here that the demonstrated feasibility of SRT for up to 10 brain lesions (48), and the serious neurocognitive sequelae of WBRT (49) should obviate use of the latter for ALK+ NSCLC, as long as potentially effective targeted treatments are still available. In contrast, tolerability is no issue with alectinib and brigatinib, which show low, single-digit rates of grade 3 adverse events, and discontinuation rates of about 10% similar to crizotinib (9,11). Even the “early-onset pulmonary events” under brigatinib appear to be less frequent in the first compared to the second line (about 3% vs. 6%) (11,19).
A first cautionary note about the “best-first” approach concerns the still immature OS data of the ALEX and ALTA-1L trials (10,11), which have not demonstrated an advantage over crizotinib yet. However, this is not an argument for waiting: given the very long survival of ALK+ NSCLC patients today, the OS advantage of the “best-first” approach would need several years to become apparent. Furthermore, the final analysis of the PROFILE-1014 study teaches that crossover can obscure the OS advantage even in case of an obviously superior treatment, such as crizotinib in the comparison against chemotherapy (40). Another cautionary note comes from the next-line treatment options following the “best-first” approach. Small series suggest limited efficacy of other second-generation ALK inhibitors, namely ceritinib (n=20) and brigatinib (n=18 cases evaluable for response), after alectinib (50,51). For brigatinib, however, in vitro and in vivo data show good activity against ALK resistance mutations, including G1202R (52), and a definite answer is eagerly awaited from an ongoing large international phase 2 trial (NCT03535740, n=103). On the other hand, the third-generation compound lorlatinib has already shown considerable activity after failure of second-generation ALK inhibitors in a global phase 2 trial, with a response rate about 40–50% for unselected patients and over 60% for the approximately 50% with detectable ALK resistance mutations (13,53). The latter represent the most frequent mechanism of acquired resistance to ALK TKI and are frequently amenable to therapy with different, more potent ALK inhibitors, the choice of which can be informed by the specific mutation present (5,43). Therefore, methods for the detection of ALK resistance mutations are rapidly gaining importance as tools for therapy guidance in ALK+ NSCLC (54,55). Currently available platforms are generally based on next-generation sequencing (NGS) of exons in the ALK kinase domain utilizing DNA obtained through tissue or liquid rebiopsies (ctDNA) at the time of disease progression (56,57). Interestingly, clinico-pathologic correlations show that the specific profile of ALK resistance mutations is shaped not only by TKI sequencing (58), but also by the ALK variant status (59), which therefore acquires therapeutic relevance in advanced ALK+ disease. However, the clinical significance of ALK fusion variants is much broader and therefore warrants a thorough discussion in the next section.
And do we care about the variants?
Diagnosis of ALK+ NSCLC is usually based on detection of ALK overexpression by immunohistochemistry or ALK translocation by fluorescence in situ hybridisation, which are common to all cases and similarly predict benefit from ALK TKI (60-62). But the actual molecular alteration, the ALK fusion itself, varies among patients, and involves the echinoderm microtubule-associated protein-like-4 (EML4) as the partner gene in about 90% of cases (63). Within EML4-ALK+ NSCLC variability also exists: approximately 30–40% of cases have the shorter EML4-ALK variant 3 (V3, E6;A20), while V1 (E13;A20) and V2 (E20;A20) are encountered about in 40% and 10% of cases, respectively (63). In routine clinical specimens, the ALK fusion variant could be typed by RT-PCR or NGS (64), but this is currently performed only in very few centers worldwide.
As already stated, these differences affect the development of ALK resistance mutations, which are encountered more frequently in V3- vs. V1-driven tumors (about 65% vs. 45%) (59). However, it is important to recognize that V3 has a relative resistance to ALK inhibitors in its native, “wild-type” state, as well. In vitro studies have demonstrated an increased IC50 of V3- vs. V1- and V2-transfected cells to first- and second-generation ALK inhibitors, which is presumably attributable to the higher stability of the V3 oncoprotein, resulting in greater accumulation and stronger ALK phosphorylation (65-67). In addition, V3 promotes microtubule stabilization through recruitment of NEK9 and NEK7 kinases, which increases cell migration and enhances metastatic potential (68). These characteristics are directly related with the special structure of V3, which is a shorter variant, devoid of the truncated EML4 β-propeller domains that reduce stability and limit interaction with the cytoskeleton in case of V1 and V2 (67).
Several clinical implications ensue from the biological differences of ALK variants. First, if the IC50 to ALK TKI varies by the ALK fusion variant per se, then the IC50 of ALK resistance mutations will not only depend on the mutation itself, but also on the ALK fusion variant that the mutation develops on. Most ALK mutation “resistograms” are generated on the V1 background (43) and do not accommodate the fact that ALK resistance mutations predominantly (in over two-thirds of cases) occur with V3 (59), which itself has a higher IC50 to ALK inhibitors than V1 (65). On the other hand, in case of a V3-based resistogram, it is important to address the shorter, V3a splice variant, which displays a higher oncogenic potential (65,66), and not V3b, which in vitro behaves similar to V1 (66,69).
Even more important is the impact of ALK fusion variants on patient outcome, which was the subject of several retrospective studies recently. PFS under ALK TKI was found longer with V1 vs. other variants (70), longer with V2 vs. other variants (71), shorter with V3 vs. V1 and V2 as analyzed by RECIST (64,65,68,72) or as time-to-next-treatment (64), and OS was also found shorter with V3 vs. V1 and V2 after a longer patient follow-up (64,68,72). At the same time, the V3-associated risk appears to be present already at diagnosis, that is before initiation of a specific treatment (73). Newly-diagnosed V3-driven tumors have a higher frequency of metastatic disease (74) and a higher number of metastatic sites when stage IV (64), consistent with the stronger ALK expression and the higher migratory capacity of V3-positive tumor cells (68,74). In line with these data, carefully controlled retrospective analyses show that V3 status is relevant for the outcome not only of double TKI-refractory patients in the third line (59), but also with first-line administration of TKI and other treatments, namely chemotherapy and cerebral radiotherapy (64). Of note, limited retrospective data suggest that other, “short” EML4-ALK variants, such as V5 (E2;A20) (65), and non-EML4-ALK fusions (75) are also associated with worse outcome, while the longer EML4-ALK V2 (E20;A20) appears to be favourable (71).
Still, a major problem for the field currently is that prospective data are lacking, because typing of ALK fusion variants has not been part of any clinical trial so far. A recent effort to complement the ALEX trial with ALK fusion variant information a posteriori, demonstrates the difficulties and limitations of this approach: typing of tissue samples using the FoundationOne panel was successful in slightly over 1/3 of cases (76). Overall, the collected data suggested a superior outcome with alectinib compared to crizotinib across the three main EML4-ALK variants V1, V2, V3, but also a trend that the benefit from alectinib, i.e., the response rate (P=0.103) and the PFS (P=0.114), might be lower in non-V1 cases. These data are still immature (they are based on the data cut-off of December 2017), and have to be interpreted with some caution, because the compared patient groups are small (n=8–25, which is lower than in previous retrospective analyses) and possibly not well balanced for other clinical and molecular parameters. However, they raise the important possibility that the ALK variant status could influence choice of the “best drug” and implementation of the “best-first” approach. Updated results of this analysis as well as the results of a planned similar analysis in the ALTA-1L trial of upfront brigatinib are eagerly awaited.
Recently, TP53 mutations were also recognized as an additional, independent molecular risk factor for earlier TKI failure and shorter OS in ALK+ NSCLC (71,72,77,78). Furthermore, detection of TP53 mutations in tissue or liquid rebiopsies at the time of disease progression in previously TP53 negative patients identifies another approximately 20% of cases with a poor outcome comparable to that with primarily TP53 mutated tumors (79). The independent and possibly synergistic effects of both the ALK fusion variant and TP53 mutations on the clinical course of ALK+ NSCLC patients (72) mean that considerable biological and clinical variability is to be expected in studies that take only one of these two factors into account. For guidance of patient management based on the molecular properties of the tumor, typing of both (likely in addition to assessment of further, still unidentified molecular features) will be required, which could for example be achieved by combined targeted RNA and DNA NGS (80).
Optimal management of higher-risk, i.e., V3+, TP53mut and particularly “double-positive” V3+TP53mut ALK+ NSCLC patients is unclear at present (1). When discussing prognosis, some reservation is warranted, especially for V3+TP53mut cases, most of which will probably not reach the 5-year landmark (72). Also, a more aggressive strategy regarding local ablative therapies should be considered at progression, otherwise some high-risk patients will fail each available ALK TKI within a few months and end up with palliative chemotherapy within a couple of years (72). More frequent radiologic surveillance, additional ctDNA monitoring (79), upfront administration of more potent ALK inhibitors and combination with experimental compounds, such as TP53-directed drugs (81), will probably also be beneficial, but requires testing in prospective clinical trials.
Conclusions
ALK+ NSCLC is currently the forerunner of “precision medicine” in thoracic oncology and a model disease for the development of novel approaches. “Best-first” treatment with upfront administration of second-generation ALK inhibitors, especially alectinib and brigatinib according to the current data, significantly delays systemic and brain progression, apparently obviates the need for early radiotherapy and is expected to increase the rate of long-term responders. Besides, the identification of EML4-ALK fusion variant 3 and TP53 mutations as independent and possibly synergistic molecular risk factor, assists selection of cases for more aggressive management and guides preclinical modeling in order to advance therapeutic options. Common denominator appears to be the lower genetic complexity of ALK+ disease, which not only facilitates the relatively favourable clinical course, but also makes study of the effects from individual molecular features easier. The growing understanding about critical molecular parameters in ALK+ disease and the increasing availability of more effective drugs continue to refine our concepts and expand the therapeutic armamentarium.
Acknowledgments
Funding: None.
Footnote
Provenance and Peer Review: This article was commissioned by the Guest Editor Nir Peled for the series dedicated to the Congress on Clinical Controversies in Lung Cancer (CCLC 2018) published in Precision Cancer Medicine. The article has undergone external peer review.
Conflicts of Interest: The author has completed the ICMJE uniform disclosure form (available at http://dx.doi.org/10.21037/pcm.2019.05.01). The series dedicated to the Congress on Clinical Controversies in Lung Cancer (CCLC 2018) was commissioned by the editorial office without any funding or sponsorship. The author reports advisory board and lecture fees from Novartis, Roche, Chugai and Boehringer Ingelheim, as well as research funding from Novartis, Roche, AstraZeneca and Takeda. The author has no other conflicts of interest to declare.
Ethical Statement: The author is accountable for all aspects of the work in ensuring that questions related to the accuracy or integrity of any part of the work are appropriately investigated and resolved.
Open Access Statement: This is an Open Access article distributed in accordance with the Creative Commons Attribution-NonCommercial-NoDerivs 4.0 International License (CC BY-NC-ND 4.0), which permits the non-commercial replication and distribution of the article with the strict proviso that no changes or edits are made and the original work is properly cited (including links to both the formal publication through the relevant DOI and the license). See: https://creativecommons.org/licenses/by-nc-nd/4.0/.
References
- Christopoulos P, Budczies J, Kirchner M, et al. Defining molecular risk in ALK+ NSCLC. Oncotarget 2019;10:3093-103. [Crossref]
- Spigel DR, Schrock AB, Fabrizio D, et al. Total mutation burden (TMB) in lung cancer (LC) and relationship with response to PD-1/PD-L1 targeted therapies. J Clin Oncol 2016;34:9017. [Crossref]
- Duruisseaux M, Besse B, Cadranel J, et al. Overall survival with crizotinib and next-generation ALK inhibitors in ALK-positive non-small-cell lung cancer (IFCT-1302 CLINALK): a French nationwide cohort retrospective study. Oncotarget 2017;8:21903-17. [Crossref] [PubMed]
- Golding B, Luu A, Jones R, et al. The function and therapeutic targeting of anaplastic lymphoma kinase (ALK) in non-small cell lung cancer (NSCLC). Mol Cancer 2018;17:52. [Crossref] [PubMed]
- Lin JJ, Riely GJ, Shaw AT. Targeting ALK: Precision Medicine Takes on Drug Resistance. Cancer Discov 2017;7:137-55. [Crossref] [PubMed]
- Solomon BJ, Mok T, Kim DW, et al. First-line crizotinib versus chemotherapy in ALK-positive lung cancer. N Engl J Med 2014;371:2167-77. [Crossref] [PubMed]
- Soria JC, Tan DSW, Chiari R, et al. First-line ceritinib versus platinum-based chemotherapy in advanced ALK-rearranged non-small-cell lung cancer (ASCEND-4): a randomised, open-label, phase 3 study. Lancet 2017;389:917-29. [Crossref] [PubMed]
- Hida T, Nokihara H, Kondo M, et al. Alectinib versus crizotinib in patients with ALK-positive non-small-cell lung cancer (J-ALEX): an open-label, randomised phase 3 trial. Lancet 2017;390:29-39. [Crossref] [PubMed]
- Peters S, Camidge DR, Shaw AT, et al. Alectinib versus Crizotinib in Untreated ALK-Positive Non-Small-Cell Lung Cancer. N Engl J Med 2017;377:829-38. [Crossref] [PubMed]
- Camidge DR, Peters S, Mok T, et al. Updated efficacy and safety data from the global phase III ALEX study of alectinib (ALC) vs crizotinib (CZ) in untreated advanced ALK+ NSCLC. J Clin Oncol 2018;36:abstr 9043.
- Camidge DR, Kim HR, Ahn MJ, et al. Brigatinib versus Crizotinib in ALK-Positive Non-Small-Cell Lung Cancer. N Engl J Med 2018;379:2027-39. [Crossref] [PubMed]
- Horn L, Infante JR, Reckamp KL, et al. Ensartinib (X-396) in ALK-Positive Non-Small Cell Lung Cancer: Results from a First-in-Human Phase I/II, Multicenter Study. Clin Cancer Res 2018;24:2771-9. [Crossref] [PubMed]
- Solomon BJ, Besse B, Bauer TM, et al. Lorlatinib in patients with ALK-positive non-small-cell lung cancer: results from a global phase 2 study. Lancet Oncol 2018;19:1654-67. [Crossref] [PubMed]
- Kim DW, Mehra R, Tan DSW, et al. Activity and safety of ceritinib in patients with ALK-rearranged non-small-cell lung cancer (ASCEND-1): updated results from the multicentre, open-label, phase 1 trial. Lancet Oncol 2016;17:452-63. [Crossref] [PubMed]
- Crinò L, Ahn MJ, De Marinis F, et al. Multicenter Phase II Study of Whole-Body and Intracranial Activity With Ceritinib in Patients With ALK-Rearranged Non-Small-Cell Lung Cancer Previously Treated With Chemotherapy and Crizotinib: Results From ASCEND-2. J Clin Oncol 2016;34:2866-73. [Crossref] [PubMed]
- Ou SHI, Ahn JS, Petris LD, et al. Alectinib in Crizotinib-Refractory ALK-Rearranged Non-Small-Cell Lung Cancer: A Phase II Global Study. J Clin Oncol 2016;34:661-8. [Crossref] [PubMed]
- Shaw AT, Gandhi L, Gadgeel S, et al. Alectinib in ALK-positive, crizotinib-resistant, non-small-cell lung cancer: a single-group, multicentre, phase 2 trial. Lancet Oncol 2016;17:234-42. [Crossref] [PubMed]
- Gettinger SN, Bazhenova LA, Langer CJ, et al. Activity and safety of brigatinib in ALK-rearranged non-small-cell lung cancer and other malignancies: a single-arm, open-label, phase 1/2 trial. Lancet Oncol 2016;17:1683-96. [Crossref] [PubMed]
- Kim DW, Tiseo M, Ahn MJ, et al. Brigatinib in Patients With Crizotinib-Refractory Anaplastic Lymphoma Kinase-Positive Non-Small-Cell Lung Cancer: A Randomized, Multicenter Phase II Trial. J Clin Oncol 2017;35:2490-8. [Crossref] [PubMed]
- Ahn M, Camidge DR, Tiseo M, et al. OA 05.05 Brigatinib in Crizotinib-Refractory ALK+ NSCLC: Updated Efficacy and Safety Results From ALTA, a Randomized Phase 2 Trial. J Thorac Oncol 2017;12:S1755-6. [Crossref]
- Brosseau S, Gounant V, Zalcman G. (J)ALEX the great: a new era in the world of ALK inhibitors. J Thorac Dis 2018;10:S2138-43. [Crossref] [PubMed]
- Reungwetwattana T, Nakagawa K, Cho BC, et al. CNS Response to Osimertinib Versus Standard Epidermal Growth Factor Receptor Tyrosine Kinase Inhibitors in Patients With Untreated EGFR-Mutated Advanced Non-Small-Cell Lung Cancer. J Clin Oncol 2018;JCO2018783118. [PubMed]
- Wu YL, Ahn MJ, Garassino MC, et al. CNS Efficacy of Osimertinib in Patients With T790M-Positive Advanced Non-Small-Cell Lung Cancer: Data From a Randomized Phase III Trial (AURA3). J Clin Oncol 2018;36:2702-9. [Crossref] [PubMed]
- Welsh JW, Komaki R, Amini A, et al. Phase II trial of erlotinib plus concurrent whole-brain radiation therapy for patients with brain metastases from non-small-cell lung cancer. J Clin Oncol 2013;31:895-902. [Crossref] [PubMed]
- Hsiao SH, Lin HC, Chou YT, et al. Impact of epidermal growth factor receptor mutations on intracranial treatment response and survival after brain metastases in lung adenocarcinoma patients. Lung Cancer 2013;81:455-61. [Crossref] [PubMed]
- Jiang T, Min W, Li Y, et al. Radiotherapy plus EGFR TKIs in non-small cell lung cancer patients with brain metastases: an update meta-analysis. Cancer Med 2016;5:1055-65. [Crossref] [PubMed]
- Gadgeel SM, Shaw AT, Govindan R, et al. Pooled Analysis of CNS Response to Alectinib in Two Studies of Pretreated Patients With ALK-Positive Non–Small-Cell Lung Cancer. J Clin Oncol 2016;34:4079-85. [Crossref] [PubMed]
- Camidge DR, Kim DW, Tiseo M, et al. Exploratory Analysis of Brigatinib Activity in Patients With Anaplastic Lymphoma Kinase-Positive Non-Small-Cell Lung Cancer and Brain Metastases in Two Clinical Trials. J Clin Oncol 2018;36:2693-701. [Crossref] [PubMed]
- Yoshida T, Oya Y, Tanaka K, et al. Clinical impact of crizotinib on central nervous system progression in ALK-positive non-small lung cancer. Lung Cancer 2016;97:43-7. [Crossref] [PubMed]
- Doebele RC, Lu X, Sumey C, et al. Oncogene status predicts patterns of metastatic spread in treatment-naive nonsmall cell lung cancer. Cancer 2012;118:4502-11. [Crossref] [PubMed]
- Rangachari D, Yamaguchi N, VanderLaan PA, et al. Brain metastases in patients with EGFR-mutated or ALK-rearranged non-small-cell lung cancers. Lung Cancer 2015;88:108-11. [Crossref] [PubMed]
- Mezquita L, Besse B. Sequencing ALK inhibitors: alectinib in crizotinib-resistant patients, a phase 2 trial by Shaw et al. J Thorac Dis 2016;8:2997-3002. [Crossref] [PubMed]
- Sullivan I, Planchard D. Editorial on the article entitled "brigatinib efficacy and safety in patients with anaplastic lymphoma kinase (ALK)-positive non-small cell lung cancer in a phase I/II trial". J Thorac Dis 2016;8:E1287-92. [Crossref] [PubMed]
- Hayashi H, Nakagawa K. Current evidence in support of the second-generation anaplastic lymphoma kinase (ALK) tyrosine kinase inhibitor alectinib for the treatment of non-small cell lung cancer positive for ALK translocation. J Thorac Dis 2016;8:E1311-6. [Crossref] [PubMed]
- NCCN Clinical Practice Guidelines in Oncology (NCCN Guidelines). Non-small cell lung cancer, version 2. 2019.
- Planchard D, Popat S, Kerr K, et al. Metastatic non-small cell lung cancer: ESMO Clinical Practice Guidelines for diagnosis, treatment and follow-up. Ann Oncol 2018;29:iv192-iv237. [Crossref] [PubMed]
- Shaw AT, Kim DW, Mehra R, et al. Ceritinib in ALK-rearranged non-small-cell lung cancer. N Engl J Med 2014;370:1189-97. [Crossref] [PubMed]
- Cortes JE. A second-generation TKI should always be used as initial therapy for CML. Blood Adv 2018;2:3653-5. [Crossref] [PubMed]
- Shaw AT, Kim DW, Nakagawa K, et al. Crizotinib versus chemotherapy in advanced ALK-positive lung cancer. N Engl J Med 2013;368:2385-94. [Crossref] [PubMed]
- Solomon BJ, Kim DW, Wu YL, et al. Final Overall Survival Analysis From a Study Comparing First-Line Crizotinib Versus Chemotherapy in ALK-Mutation-Positive Non-Small-Cell Lung Cancer. J Clin Oncol 2018;36:2251-8. [Crossref] [PubMed]
- Cheung-Ong K, Giaever G, Nislow C. DNA-damaging agents in cancer chemotherapy: serendipity and chemical biology. Chem Biol 2013;20:648-59. [Crossref] [PubMed]
- Sotillo R, Schvartzman JM, Socci ND, et al. Mad2-induced chromosome instability leads to lung tumour relapse after oncogene withdrawal. Nature 2010;464:436-40. [Crossref] [PubMed]
- Gainor JF, Dardaei L, Yoda S, et al. Molecular Mechanisms of Resistance to First- and Second-Generation ALK Inhibitors in ALK-Rearranged Lung Cancer. Cancer Discov 2016;6:1118-33. [Crossref] [PubMed]
- Westover D, Zugazagoitia J, Cho BC, et al. Mechanisms of acquired resistance to first- and second-generation EGFR tyrosine kinase inhibitors. Ann Oncol 2018;29:i10-9. [Crossref] [PubMed]
- Magnuson WJ, Lester-Coll NH, Wu AJ, et al. Management of Brain Metastases in Tyrosine Kinase Inhibitor-Naïve Epidermal Growth Factor Receptor-Mutant Non-Small-Cell Lung Cancer: A Retrospective Multi-Institutional Analysis. J Clin Oncol 2017;35:1070-7. [Crossref] [PubMed]
- Lin JJ, Jiang GY, Joshipura N, et al. Efficacy of Alectinib in Patients with ALK-Positive NSCLC and Symptomatic or Large CNS Metastases. J Thorac Oncol 2019;14:683-90. [Crossref] [PubMed]
- Stephens SJ, Moravan MJ, Salama JK. Managing Patients With Oligometastatic Non-Small-Cell Lung Cancer. J Oncol Pract 2018;14:23-31. [Crossref] [PubMed]
- Yamamoto M, Serizawa T, Shuto T, et al. Stereotactic radiosurgery for patients with multiple brain metastases (JLGK0901): a multi-institutional prospective observational study. Lancet Oncol 2014;15:387-95. [Crossref] [PubMed]
- Tallet AV, Azria D, Barlesi F, et al. Neurocognitive function impairment after whole brain radiotherapy for brain metastases: actual assessment. Radiat Oncol 2012;7:77. [Crossref] [PubMed]
- Hida T, Seto T, Horinouchi H, et al. Phase II study of ceritinib in alectinib-pretreated patients with anaplastic lymphoma kinase-rearranged metastatic non-small-cell lung cancer in Japan: ASCEND-9. Cancer Sci 2018;109:2863-72. [Crossref] [PubMed]
- Lin JJ, Zhu VW, Schoenfeld AJ, et al. Brigatinib in Patients With Alectinib-Refractory ALK-Positive NSCLC. J Thorac Oncol 2018;13:1530-8. [Crossref] [PubMed]
- Zhang S, Anjum R, Squillace R, et al. The Potent ALK Inhibitor Brigatinib (AP26113) Overcomes Mechanisms of Resistance to First- and Second-Generation ALK Inhibitors in Preclinical Models. Clin Cancer Res 2016;22:5527-38. [Crossref] [PubMed]
- Shaw AT, Solomon BJ, Besse B, et al. ALK Resistance Mutations and Efficacy of Lorlatinib in Advanced Anaplastic Lymphoma Kinase-Positive Non-Small-Cell Lung Cancer. J Clin Oncol 2019;JCO1802236. [Crossref] [PubMed]
- McCoach CE, Blakely CM, Banks KC, et al. Clinical Utility of Cell-Free DNA for the Detection of ALK Fusions and Genomic Mechanisms of ALK Inhibitor Resistance in Non-Small Cell Lung Cancer. Clin Cancer Res 2018;24:2758-70. [Crossref] [PubMed]
- Dagogo-Jack I, Brannon AR, Ferris LA, et al. Tracking the Evolution of Resistance to ALK Tyrosine Kinase Inhibitors through Longitudinal Analysis of Circulating Tumor DNA. JCO Precis Oncol 2018;2018.
- Chen Y, Guo W, Fan J, et al. The applications of liquid biopsy in resistance surveillance of anaplastic lymphoma kinase inhibitor. Cancer Manag Res 2017;9:801-11. [Crossref] [PubMed]
- Volckmar AL, Sultmann H, Riediger A, et al. A field guide for cancer diagnostics using cell-free DNA: From principles to practice and clinical applications. Genes Chromosomes Cancer 2018;57:123-39. [Crossref] [PubMed]
- Yoda S, Lin JJ, Lawrence MS, et al. Sequential ALK Inhibitors Can Select for Lorlatinib-Resistant Compound ALK Mutations in ALK-Positive Lung Cancer. Cancer Discov 2018;8:714-29. [Crossref] [PubMed]
- Lin JJ, Zhu VW, Yoda S, et al. Impact of EML4-ALK Variant on Resistance Mechanisms and Clinical Outcomes in ALK-Positive Lung Cancer. J Clin Oncol 2018;36:1199-206. [Crossref] [PubMed]
- Kalemkerian GP, Narula N, Kennedy EB, et al. Molecular Testing Guideline for the Selection of Patients With Lung Cancer for Treatment With Targeted Tyrosine Kinase Inhibitors: American Society of Clinical Oncology Endorsement of the College of American Pathologists/International Association for the Study of Lung Cancer/Association for Molecular Pathology Clinical Practice Guideline Update. J Clin Oncol 2018;36:911-9. [Crossref] [PubMed]
- Blackhall FH, Peters S, Bubendorf L, et al. Prevalence and clinical outcomes for patients with ALK-positive resected stage I to III adenocarcinoma: results from the European Thoracic Oncology Platform Lungscape Project. J Clin Oncol 2014;32:2780-7. [Crossref] [PubMed]
- Gelsomino F, Rossi G, Tiseo M. Clinical implications and future perspectives in testing non-small cell lung cancer (NSCLC) for anaplastic lymphoma kinase (ALK) gene rearrangements. J Thorac Dis 2015;7:220-3. [PubMed]
- Sabir SR, Yeoh S, Jackson G, et al. EML4-ALK Variants: Biological and Molecular Properties, and the Implications for Patients. Cancers (Basel) 2017;9:118. [Crossref] [PubMed]
- Christopoulos P, Endris V, Bozorgmehr F, et al. EML4-ALK fusion variant V3 is a high-risk feature conferring accelerated metastatic spread, early treatment failure and worse overall survival in ALK+ NSCLC. Int J Cancer 2018;142:2589-98. [Crossref] [PubMed]
- Woo CG, Seo S, Kim SW, et al. Differential protein stability and clinical responses of EML4-ALK fusion variants to various ALK inhibitors in advanced ALK-rearranged non-small cell lung cancer. Ann Oncol 2017;28:791-7. [PubMed]
- Heuckmann JM, Balke-Want H, Malchers F, et al. Differential protein stability and ALK inhibitor sensitivity of EML4-ALK fusion variants. Clin Cancer Res 2012;18:4682-90. [Crossref] [PubMed]
- Richards MW, Law EW, Rennalls LP, et al. Crystal structure of EML1 reveals the basis for Hsp90 dependence of oncogenic EML4-ALK by disruption of an atypical beta-propeller domain. Proc Natl Acad Sci U S A 2014;111:5195-200. [Crossref] [PubMed]
- O’Regan L, Barone G, Adib R, et al. EML4-ALK V3 drives cell migration through NEK9 and NEK7 kinases in non-small-cell lung cancer. bioRxiv 2019;567305.
- Childress MA, Himmelberg SM, Chen H, et al. ALK Fusion Partners Impact Response to ALK Inhibition: Differential Effects on Sensitivity, Cellular Phenotypes, and Biochemical Properties. Mol Cancer Res 2018;16:1724-36. [Crossref] [PubMed]
- Yoshida T, Oya Y, Tanaka K, et al. Differential Crizotinib Response Duration Among ALK Fusion Variants in ALK-Positive Non-Small-Cell Lung Cancer. J Clin Oncol 2016;34:3383-9. [Crossref] [PubMed]
- Li Y, Zhang T, Zhang J, et al. Response to crizotinib in advanced ALK-rearranged non-small cell lung cancers with different ALK-fusion variants. Lung Cancer 2018;118:128-33. [Crossref] [PubMed]
- Christopoulos P, Kirchner M, Bozorgmehr F, et al. Identification of a highly lethal V3+TP53+ subset in ALK+ lung adenocarcinoma. Int J Cancer 2019;144:190-9. [Crossref] [PubMed]
- Christopoulos P, Kirchner M, Endris V, et al. EML4-ALK V3, treatment resistance, and survival: refining the diagnosis of ALK+ NSCLC. J Thorac Dis 2018;10:S1989-91. [Crossref] [PubMed]
- Noh KW, Lee MS, Lee SE, et al. Molecular breakdown: a comprehensive view of anaplastic lymphoma kinase (ALK)-rearranged non-small cell lung cancer. J Pathol 2017;243:307-19. [Crossref] [PubMed]
- Rosenbaum JN, Bloom R, Forys JT, et al. Genomic heterogeneity of ALK fusion breakpoints in non-small-cell lung cancer. Mod Pathol 2018;31:791-808. [Crossref] [PubMed]
- Camidge DR, Dziadziuszko R, Peters S, et al. Updated Efficacy and Safety Data and Impact of the EML4-ALK Fusion Variant on the Efficacy of Alectinib in Untreated ALK-positive Advanced Non-small-cell Lung Cancer in the Global Phase III ALEX Study. J Thorac Oncol 2019; [Epub ahead of print]. [Crossref] [PubMed]
- Kron A, Alidousty C, Scheffler M, et al. Impact of TP53 mutation status on systemic treatment outcome in ALK-rearranged non-small-cell lung cancer. Ann Oncol 2018;29:2068-75. [Crossref] [PubMed]
- Wang WX, Xu CW, Chen YP, et al. TP53 mutations predict for poor survival in ALK rearrangement lung adenocarcinoma patients treated with crizotinib. J Thorac Dis 2018;10:2991-8. [Crossref] [PubMed]
- Christopoulos P, Dietz S, Kirchner M, et al. Detection of TP53 Mutations in Tissue or Liquid Rebiopsies at Progression Identifies ALK+ Lung Cancer Patients with Poor Survival. Cancers (Basel) 2019;11. [PubMed]
- Volckmar AL, Leichsenring J, Kirchner M, et al. Combined targeted DNA and RNA sequencing of advanced NSCLC in routine molecular diagnostics: Analysis of the first 3,000 Heidelberg cases. Int J Cancer 2019; [Epub ahead of print]. [Crossref] [PubMed]
- Bykov VJN, Eriksson SE, Bianchi J, et al. Targeting mutant p53 for efficient cancer therapy. Nat Rev Cancer 2018;18:89-102. [Crossref] [PubMed]
Cite this article as: Christopoulos P. ALK disease: best first or later, and do we care about variants? Precis Cancer Med 2019;2:16.