Genetic and non-genetic resistance mechanisms in non-small cell lung cancer harboring (non-ALK) oncogenic fusions
Introduction
Non-small cell lung cancer (NSCLC) accounts for 85% of lung cancer cases and is still the main cause of cancer-related deaths worldwide. However, the prognosis of NSCLC patients has improved remarkably over the last decade, primarily attributable to the introduction of immunotherapy and targeted therapies. In this light, approximately 25–30% of NSCLC cases present a molecular driver that may nowadays predict the response to selective therapies. This therapeutic evolution has resulted in a substantial improvement in the survival of these patients, going from less than 2 years up to 5 or even more years (1). Against several molecular alterations, including mutations of EGFR, BRAF p.V600 and KRAS p.G12C, HER2, alterations leading to the skipping of MET exon 14, and gene fusions or rearrangements of ALK, ROS1, NTRK, and RET, target therapies are nowadays available (2). Furthermore, ongoing research into novel gene alterations holds the promise of broadening our understanding of oncogene-driven NSCLC. Gene fusions represent a subtype of molecular alterations arising from chromosomal rearrangements or splicing errors occurring during transcription. These events result in the production of chimeric proteins, which ultimately promote multiple mechanisms facilitating the proliferation of cancer cells. Historically, gene fusions have been predominantly associated with hematologic malignancies (i.e., BCR-ABL fusion in chronic myeloid leukemia) (3). However, with the advancements in molecular biology techniques, and with the advent of next-generation sequencing (NGS) in particular, a more extensive array of gene rearrangements and fusions has also come to the forefront in the context of solid tumors. This expanded knowledge has shed light on their role in cancer development and potential response to targeted therapies (4,5).
In the expanding panorama of oncogene-driven NSCLC, gene fusions account for approximately 5–7% in some extensive analyses of patients affected by NSCLC (6,7). These alterations are often examined through fluorescence in situ hybridization (FISH), immunohistochemistry (IHC), or real-time polymerase chain reaction (RT-PCR) methodologies, with the possibility of missing a relevant number of cases, due to their low sensitivity and specificity in detecting gene fusions. In contrast, RNA-based NGS can analyze a broad spectrum of genes, as well as their transcripts, providing enhanced precision and sensitivity in this setting (8). In recent years, significant advancements in gene fusion-targeted therapies yielded encouraging efficacy (9). Nevertheless, resistance to these treatments inevitably occurs, leading to disease progression. Therefore, it is important to examine the potential resistance mechanisms to enable the development of precise therapeutic interventions.
While ALK rearrangements have undergone extensive investigations, resulting in the development of sequential strategies for patients experiencing progression after first-line treatments (9), the post-progression management of patients harboring gene fusions such as ROS1, RET, or NTRK remains an active area of research. In these cases, therapeutic options upon disease progression remain limited, often rendering the selection of subsequent lines of treatment a complex and challenging effort.
This review aims to assess the current knowledge about genetic and non-genetic resistance mechanisms to tyrosine kinase inhibitors (TKIs) in NSCLC harboring non-ALK gene fusions. In addition, we report the most recently identified fusions and their potential future role in clinical practice.
ROS1 fusions
ROS1 is a proto-oncogene encoding for a tyrosine kinase with high homology with ALK, promoting signal transduction for cell growth and survival. Among patients affected by NSCLC, ROS1 fusions occur in 1–2% of cases, mainly with adenocarcinoma histology, young age, and non-smoker status (10).
Several ROS1 fusion partners have been identified in NSCLC patients, with the most frequent being CD74 (44%), followed by EZR (16%), SDC4 (14%), and SLC34A2 (10%) (11). These fusions lead to the loss of the extracellular domain, resulting in ligand-independent activation of downstream signaling pathways, such as mitogen-activated protein kinase (MAPK), phosphoinositide 3-kinases (PI3K), janus kinases-signal transducer and activator of transcription proteins (JAK-STAT) (11). ROS1 inhibitors, including crizotinib and entrectinib, exert their effects by binding to the active form of the ROS1 kinase. In contrast, ongoing research is focused on novel type II inhibitors, such as foretinib, designed to specifically interact with the inactive kinase state (11).
Crizotinib is a first-generation ALK TKI, which also showed efficacy in ROS1-rearranged NSCLC. Based on the results of the phase Ib PROFILE 1001 trial, with a 72% overall response rate (ORR), median duration of response (mDOR) of 24.7 months, and median overall survival (mOS) of 51.4 months in treatment-naïve patients (12), confirmed by the subsequent phase II METROS (13) and EUCROSS (14) trials, crizotinib become one of the recommended first-line treatments for ROS1-rearranged NSCLC.
Entrectinib, an Food and Drug Administration (FDA) and European Medicines Agency (EMA)-approved first-line alternative to crizotinib, is a TRK A/B/C, ALK, and ROS1 inhibitor, which demonstrated a 40-times higher efficacy in in vitro models compared to crizotinib (15). Its efficacy was assessed in an updated analysis of the three ongoing phase I or II trials, ALKA-372-001, STARTRK-1, and STARTRK-2. This analysis included 168 patients with advanced NSCLC harboring ROS1 rearrangements, treatment-naive. The ORR was 68%, the median progression-free survival (mPFS) was 15.7 months, and the mOS was 47.8 months in the overall patient population. Among the subset of patients with baseline central nervous system (CNS) metastases (n=25), the intracranial ORR was 80%, the mPFS was 11.8 months, and the mOS was 28.3 months (16). Based on these results, entrectinib is preferred over crizotinib in patients with brain metastases (17).
Ceritinib is a second-generation ALK and ROS1 inhibitor with efficacy in ROS1 TKI-naïve patients, but its use is limited due to an unfavorable gastrointestinal toxicity profile (18). On the other hand, Lorlatinib showed efficacy both in treatment-naïve patients (ORR 62% and PFS 21 months, with an intracranial ORR of 64%) and in patients who had previously received crizotinib (ORR 35% and mPFS 8.5 months) (19). However, neither ceritinib nor lorlatinib has still received approval from EMA (17).
Next-generation TKIs have been developed but no mature data to endorse their use in daily practice are available. In the phase I/II TRIDENT-1 trial, repotrectinib showed 79% of confirmed responses in non-pretreated patients with a mDOR of 34.1 months while in a similar population (20), taletrectinib had a 94% ORR in a phase II trial (21). Of note, both showed efficacy in subsequent lines (ORR 38% and 55%, respectively) (20,21).
Intrinsic resistance mechanisms
Among ROS1-intrinsic resistance mechanisms, ROS1 point mutations have been widely described, especially in crizotinib-resistant patients. ROS1 G2032R is the most common mutation in patients experiencing disease progression on crizotinib, occurring in 30–40% of cases (22). The G2032R mutation consists of a glycine-to-arginine substitution at codon 2032 in the ROS1 kinase domain, leading to resistance against crizotinib, ceritinib, and entrectinib by disrupting drug binding through steric interference (23). Moreover, epithelial-mesenchymal transition (EMT) and invasion are enhanced by this mutation through the activation of Twist-1, which promotes EMT through the suppression of E-cadherin expression (24,25). Many other point mutations have been described as resistance mechanisms to crizotinib, with variable incidence according to different retrospective studies. ROS1 L1951R and S1986Y/F, similarly to ROS1 G2032R, prevent drug-receptor binding with a steric mechanism in the solvent front site or in the αC helix, as well as D2033N, which occurred in 6% of cases in a specimen analysis of 39 patients (26).
Interestingly, only 15% of patients with intracranial-only progression harbored resistance point mutations, suggesting a non-genomic-based, but intrinsic resistance mechanism due to the poor blood-brain barrier penetration of crizotinib (26). This hypothesis is supported by another retrospective study on 33 ROS1-positive patients, with a 47% intracranial-only progression to crizotinib (27).
Point mutations leading to resistance to other TKIs have also been described. G2032R remains the most common resistance mechanism to lorlatinib and entrectinib, occurring in about 30% of cases (28), while repotrectinib and taletrectinib demonstrated activity also against this alteration (11). Similarly, ROS1 L2086F prevents the binding of lorlatinib and entrectinib (29), but cabozantinib and foretinib activity may be retained, according to preclinical models (28). Interestingly, ROS1 L2086F is analogous to ALK L1256F, conferring resistance to lorlatinib and crizotinib in ALK fusion-positive NSCLC. Compound ROS1 point mutations in the same sample represent a challenging situation with potential cross-resistance to available TKIs (28,30,31).
Extrinsic resistance mechanisms
A significant proportion of patients, ranging from 30 to 50%, did not harbor ROS1 point mutations, suggesting that also ROS1-extrinsic resistance mechanisms may emerge, through the activation of specific downstream pathways. In the context of ROS1-extrinsic resistance mechanisms, BRAF V600E mutations have been reported after treatment with crizotinib (32). In this sense, a case report has outlined a clinical benefit from the combinatorial administration of ROS1 and anti-BRAF agents in a ROS1-rearranged patient harboring BRAF V600E mutation (33).
Moreover, KRAS G12D mutations have been reported upon progression to crizotinib (34), alongside several KRAS and NRAS alterations that activate the MAPK pathway (28). An analysis of 35 ROS1-rearranged patients revealed that MAPK pathway activation was associated with a poorer prognosis and innate resistance to ROS1 TKIs (35). In another study involving cell lines obtained from a patient’s sample after progression to crizotinib, a shift towards an EGFR-driven cell growth was observed (36).
A single case report described the development of resistance to crizotinib attributed to the emergence of mutations within the C-kit gene (37). MET amplification has been identified in at least two separate studies through tissue rebiopsy, following both lorlatinib (29) and crizotinib (28). This amplification was also confirmed in patient-derived cell lines following progression to entrectinib (38) (Table 1, Figure 1).
Table 1
Gene fusions | Drug | Main resistance mechanisms | Treatments with reported preliminary activity |
---|---|---|---|
ROS1 | Crizotinib | ROS1 mutations | |
• G2032R | Repotrectinib, taletrectinib | ||
BRAF mutations | |||
• V600E | ROS1 TKIs + anti-BRAF agents | ||
Entrectinib | ROS1 mutations | ||
• G2032R | Repotrectinib, taletrectinib | ||
Lorlatinib | ROS1 mutations | ||
• G2032R | Repotrectinib, taletrectinib | ||
BRAF mutations | |||
• V600E | ROS1 TKIs + anti-BRAF agents | ||
NTRK | Entrectinib | NTRK1 mutations | |
• G595R | Selitrectinib, repotrectinib | ||
• G667S/C | Type II inhibitors | ||
NTRK3 mutations | |||
• G623R | Selitrectinib, repotrectinib | ||
IGF1R mutations | Cabozantinib + IGFR inhibitor (OSI-906) | ||
Larotrectinib | NTRK1 mutations | ||
• G595R | Selitrectinib, repotrectinib | ||
• G667S/C | Type II inhibitors | ||
NTRK3 mutations | |||
• G623R | Selitrectinib, repotrectinib | ||
RET | RET mutation | ||
Selpercatinib, pralsetinib | • G810R/S/C/V | TPX-0046, Loxo-260, vepafestinib, EP0031, APS03118 | |
• Y806C/N | |||
• V804L/M | |||
MET amplification | Selpercatinib + MET inhibitor |
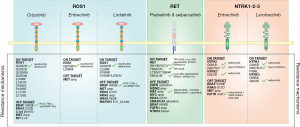
NTRK fusions
NTRK gene family includes NTRK1, NTRK2, and NTRK3, encoding for TRK family proteins TRKA, TRKB, and TRKC, respectively, which are transmembrane tyrosine kinase receptors. TRKA selectively binds to nerve growth factor (NGF), and TRKB binds to brain-derived neurotrophic factor (BDNF) (39). TRKC, on the other hand, selectively binds to neurotrophin 3 (NT-3), which can also bind to the other two members of the TRK family but with decreased activity (40). After the receptor-ligand binding, the dimerization of the TK domains elicits multiple intracellular signaling pathways, activating phospholipase-Cᵧ, MAPK, and PI3K with the final effect of promoting cell growth, neural differentiation, cell metabolism, and survival to apoptosis (41).
NTRK fusions drive the oncogenesis of multiple solid tumors and occur in lung cancer in about 0.2% of cases (42). Approximately 88 fusion partners have been identified, with different distributions among solid tumors. In lung cancer, the most common NTRK1 fusion partner is TPM3, followed by many other partners such as SQSTM1, TPR, IRF2BP2, BCL9, LMNA, PHF20 and CD74 (43). TV6 and SQSTM1 were identified as NTRK3 fusion partners. In NSCLC, NTRK fusions are usually mutually exclusive with other driver fusions and mutations, while co-occurring mutations of TP53, RB1, and NF1 have been reported (44). A younger age and a non-smoking history typically characterize patients with NSCLC harboring NTRK fusions. However, it is worth noting that this genetic alteration has also been reported in older patients and smokers (45).
FDA granted accelerated approval to two first-generation selective TRK inhibitors, larotrectinib and entrectinib, as tumor-agnostic drugs (46). Larotrectinib, a highly selective panTRK inhibitor with high CNS activity, has been investigated in three phase I/II clinical trials (NCT02122913, NCT02637687, and NCT02576431) involving patients with solid tumors harboring NTRK fusions. Among patients with NSCLC, ORR was 74% and a mPFS of 33.0 months (47). Entrectinib is a multitarget inhibitor with activity on ALK, ROS1, TRK A/B/C, and a high CNS penetration. Its therapeutic potential has been assessed through three phase I/II trials, namely STARTRK-2, STARTRK-1, and ALKA 372-001. An integrated analysis including these trials revealed a remarkable 64.5% ORR, within the NSCLC cohort (48). Additionally, the mDOR was 27.1 months, while the mPFS reached 20.8 months (48).
Intrinsic resistance mechanisms
The most common on target mechanisms involve point mutations occurring in the ATP binding pocket, particularly in the solvent front, gatekeeper region, and xDFG motif, preventing the binding of TRK inhibitors to the TK domain (49). Several point mutations have been reported among different histologies, mainly in case reports and in vitro studies on patient-derived cell lines. The TRKA solvent-front mutation G595R and the gatekeeper mutation G667S/C were both identified as resistance mechanisms to entrectinib and larotrectinib in colorectal cancer and in NSCLC (50,51), as well as the NTRK3 G623R mutation was reported in a mammary analogue secretory carcinoma (52). Additionally, a novel gatekeeper region mutation in TRKA, an xDFG mutation in NTRK3 (G696A), and a solvent front mutation in NTRK3 (G623R) have been described as potential resistance mechanisms to larotrectinib (53).
Next-generation TRK inhibitors have been developed to overcome TRK on-target resistance mechanisms. Selitrectinib is a TRK-selective inhibitor, which showed significant in vitro activity against NTRK fusions with resistance mutations, such as TRKA G595R and TRKC G623R, with an ORR of 45% (9/20) in patients with TRK kinase mutation (54). Interestingly, no activity was demonstrated in NTRK resistance due to activation of downstream pathways (ORR 0%) (54). Repotrectinib is a next-generation ALK, ROS1, and NTRK inhibitor with preclinical activity both on the “wildtype” TRK fusions and on the solvent-front mutations TRKA G595R, TRKB G639R and TRKC G623R (55). The phase I/II trial TRIDENT-1 evaluated repotrectinib in patients with advanced solid tumors harboring ROS1, ALK or NTRK fusions, both treatment-naïve and pretreated, and preliminary data showed a significant ORR in both populations, including an efficient CNS activity (ORR 33% in TKI-pretreated patients with CNS metastases) (56). Moreover, repotrectinib demonstrated activity against TRKA G595R/F589L compound mutation in cis in preclinical Ba/F3 cells (55). Taletrectinib is a ROS1 and NTRK inhibitor demonstrating promising preclinical activity against the G595R mutation in NTRK1. Nevertheless, resistance was observed mainly due to NTRK1 G667C mutation in the xDFG region (57). Although clinical data are available for the ROS1 population, evidence for NTRK fusion-positive NSCLC remains limited and necessitates further investigations (58).
Resistance mediated by mutations in the xDFG region remains an issue, as next-generation TRK inhibitors selitrectinib, repotrectinib, and taletrectinib activity is impaired in this subtype through steric hindrance (49). A putative strategy to overcome this mechanism could be using type II inhibitors such as altiratinib, cabozantinib, and foretinib which showed activity against NTRK1 G667 in preclinical data (57-59).
Extrinsic resistance mechanisms
As an off-target mechanism, downstream activation of the MAPK pathway was observed in NTRK1 fusion-positive models of pancreatic cancer and lung cancer treated with entrectinib. In several mouse models driven by an NTRK1 fusion, the vertical combination of TRKA and MEK inhibition has demonstrated the ability to significantly delay the onset of acquired resistance (60). Additionally, BRAF V600E, KRAS G12D, and MET amplification were also observed, suggesting a hint for future investigations about a potential combinatorial strategy to overcome this resistance (49).
Insulin-growth factor 1 (IGF1) receptor mutations were identified in TPM3-NTRK1-transformed Ba/F3 cells as a bypass pathway-mediated resistance, and a combination of cabozantinib and an IGFR inhibitor was able to overcome this mechanism (61).
Interestingly, in a study conducted on NTRK1 rearranged cell cultures, a various number of growth factors influenced response to entrectinib. In particular, high levels of hepatocyte growth factor (HGF) induced complete unresponsiveness to the drug by activating the MET receptor. Of note, in this preclinical model, the MET inhibitor capmatinib reversed this resistance (62) (Table 1, Figure 1).
RET fusions
Approximately 2–3% of patients with NSCLC have RET rearrangements, predominantly associated with a never or light smoking history and adenocarcinoma histology. RET maps to chromosome 10q11.2 and encodes a receptor tyrosine kinase consisting of an extracellular domain (containing four cadherin-like repeats, a calcium-binding site, and a cysteine-rich region), a transmembrane domain and an intracellular kinase domain (63). Ligand binding activates RET by forming homodimers and autophosphorylation of the TK domains. The phosphotyrosine residues serve as docking sites for the SH2 domains of several signaling molecules that activate downstream pathways (RAS/MAPK/ERK, PI3K/AKT, and JAK/STAT) associated with cellular proliferation, migration, and differentiation (64). Two high selective TKIs targeting RET, pralsetinib and selpercatinib, have significantly transformed the therapeutic landscape for tumors harboring RET alterations, establishing themselves as the standard second-line treatment for advanced NSCLC. Updated findings from the LIBRETTO-001 trial demonstrated that among patients previously treated with platinum chemotherapy (n=247), the ORR was 61%, with a mPFS of 24.9 months while in treatment-naïve patients (n=69), the ORR reached 84%, accompanied by an mPFS of 22 months (65).
Further insights into pralsetinib safety and efficacy in patients with RET fusion-positive NSCLC, as part of the ARROW trial, were recently published. Specifically, the ORR was 59% in patients previously treated with platinum-based chemotherapy and increased to 72% in treatment-naïve patients (66). The results of the LIBRETTO-431 trial, which compared selpercatinib as a frontline treatment versus standard chemoimmunotherapy (combination performed in 81% of patients in the control arm) are expected to change the first-line standard approach for these patients (67). Selpercatinib demonstrated a mPFS of 24.8 months compared to 11.2 months with chemoimmunotherapy. The ORR was 84% with selpercatinib and 65% with the control treatment. Importantly, selpercatinib exhibited excellent intracranial activity and ability to prevent CNS metastatic spread. Among patients with baseline brain metastases, the intracranial response occurred in 82% of those in the selpercatinib group, surpassing the 58% observed in the control group. These comprehensive findings contribute valuable insights into the evolving landscape of targeted therapies for RET-altered NSCLC, highlighting the efficacy, safety, and intracranial activity of selective RET inhibitors.
Intrinsic resistance mechanisms
Many cases of mutations can be traced back to those occurring at the gatekeeper residue in the hinge region of the kinase, directly preventing or weakening the interaction with the inhibitory molecule. In contrast, for RET-selective drugs, crystal structure studies of RET-kinase-selpercatinib and RET-kinase-pralsetinib complexes have shown that they dock one end in the front cleft of the drug-binding pocket without inserting through the gate; this novel binding mode is responsible for their high affinity and their ability to avoid disruption of the gatekeeper mutations (64). However, it also leaves them vulnerable to non-gatekeeper mutations, such as solvent front mutations which reside in the solvent front of the adenosine triphosphate-binding pocket in the catalytic region of the kinase domain (64).
In a retrospective study, the emergence of potential resistance mechanisms was evaluated through the execution of 105 biopsies in 89 patients exhibiting progression on RET TKIs, with RET mutations occurring in 13% of cases (68). The earliest reported resistance mechanism was the RET G810 solvent front mutation, which causes steric interference with TKIs binding and has been identified in 7–11% of post-progression biopsies. The Y806C/N mutations at the hinge region of RET also cause resistance to selpercatinib and pralsetinib. The L730V/I mutations at the roof region have been identified in pralsetinib-resistant samples, but are predicted to remain sensitive to selpercatinib (69). Regarding RET solvent front-mediated resistance, novel RET TKIs are currently under investigation. Vepafestinib, TPX-0046, LOXO-260, EP0031, and APS03118 are advanced pharmaceutical compounds that selectively inhibit RET, demonstrating potent inhibitory effects against RET solvent front mutations and gatekeeper mutations (70-73).
Extrinsic resistance mechanisms
Off target resistance alterations are the main cause of resistance in RET TKIs-treated patients. Regarding primary resistance mechanisms, MET activation was found to be a potentially targetable mediator of resistance to RET-directed therapy in a retrospective study with selpercatinib. In four cases with an unusually short benefit from selpercatinib, the combination with the MET/ALK/ROS1 inhibitor crizotinib resulted in prolonged benefit. In three out of the four cases, MET amplification was present prior to selpercatinib exposure, indicating its potential contribute as an intrinsic tumoral resistance mechanism. Furthermore, MET amplification can also represent an acquired/secondary mechanism of resistance to RET inhibitors. MET amplification was observed in 15% of 23 tumor and liquid biopsies from patients with advanced RET fusion-positive NSCLC treated with pralsetinib and selpercatinib (74-79). These results are further supported and validated by a large retrospective study (n=89) identifying the presence of MET amplification in 12% of TKIs pretreated patients (68).
The role of KRAS and SMARCA4 co-mutations in mediating primary resistance was suggested in a retrospective NGS analysis from 95 patients with RET fusion-positive NSCLC treated with a RET inhibitor (80). In 23% of patients, primary resistance was noted (disease progression within 6 months), and KRAS and SMARCA4 mutations were identified only in poor responders (74-79). MET/MYC amplifications were also identified after RET TKIs in patients with RET fusion-positive NSCLC (81). However, for a substantial proportion (40–80%) of cases, mechanisms of resistance to RET-selective TKIs remain still undetermined (69). In this light, considering also the expected treatment shift related to the positive results of the LIBRETTO-431 trial, the deep characterization of the landscape of resistance mechanisms to RET TKIs is crucial to further improve patients’ prognosis (Table 1, Figure 1).
Novel and emerging fusions
Recently, with the advent of NGS techniques, particularly RNA-based platforms, novel fusions emerged that may have a role in NSCLC and may be targeted in the future.
Neuregulin 1 (NRG1) is a member of the epidermal growth factor (EGF) ligand family, and its signal is transduced through the HER/ERBB family TKR (82). In lung cancer, NRG1 gene fusions were more common in never-smokers (57%), in non-metastatic patients (71%), and in invasive mucinous adenocarcinoma. Patients harboring these alterations showed lower benefits from first-line chemotherapy, chemoimmunotherapy, and immunotherapy alone. In contrast, retrospective data on patients treated with afatinib (20 out of 110) showed an encouraging activity (ORR 25% and mPFS 2.8 months), regardless of the fusion partner, suggesting that targeting the HER/ERBB pathway may be an effective strategy (83). Many trials are ongoing to investigate anti-HER TKIs and monoclonal antibodies against this alteration as an agnostic target (84). In particular, zenocutuzumab (an anti-HER3 antibody) showed a 29% ORR regardless of tumor histology and a 42% ORR in pancreatic cancer (85).
FGFR family includes FGFR1, FGFR2, FGFR3, FGFR4, and several fibroblast growth factor ligands (86). FGFR fusions occurs in approximately 1% of lung cancer patients (2–3% in squamous histology) (87). Some early data are available on FGFR inhibitors. A phase II trial of dovitinib (an FGFR inhibitor) in 26 patients with squamous cell cancer of the lung showed a mDOR of 2.5 months and an ORR of 11.5%, with a 50% of disease control rate (DCR) and 3 patients with a partial response (PR) (88). In two case reports, erdafitinib (an FGFR inhibitor) showed an interesting activity with 11 months of DCR (89).
MET fusions are almost anecdotical, as only some case reports and a nine-patients cohort are available in this regard. Crizotinib showed significant activity in two patients with HLA-DRB1-MET fusion and MET-ATXN7L1, respectively (90,91). In the nine patients’ cohort, four were treated with MET TKI, with two partial responses, one stable disease, and one progressive disease. In this report, one patient had a BRAF V600E mutation as an acquired resistance mechanism (92).
EGFR, BRAF, and HER2 fusions may occur in a very low percentage of patients with NSCLC, and data are missing about the optimal treatment of these cases, even if some evidence is reported with the use of erlotinib for EGFR-RAD51 and EGFR-SEPT14 fusions (9,93) (Table 2).
Table 2
Fusion | Drugs | Drug characteristics | Setting | Primary endpoints | Trial identifier |
---|---|---|---|---|---|
NRG1 | Zenocutuzumab | Anti-HER3 Ab | Advanced solid tumors | ORR, DoR | NCT02912949; phase I/II; (recruiting) |
HMBD-001 | Anti-HER3 Ab | Advanced solid tumors | Safety, ORR | NCT05919537; phase Ib; (recruiting) | |
Seribantumab | Anti-HER3 Ab | Advanced solid tumors | ORR | NCT04383210; phase II; active, not recruiting | |
FGFR | Pemigatinib | FGFR inhibitor | Advanced NSCLC | ORR | NCT05210946; recruiting |
Sintilimab + pemigatinib | Anti-PD1 + FGFR inhibitor | Advanced NSCLC | ORR | NCT05004974; phase II; recruiting | |
CPL304110 | FGFR inhibitor | Advanced solid tumors | MTD, safety | NCT04149691; phase I; recruiting | |
MET | Elzovantinib (TPX-0022) | MET/CSF1R/SRC inhibitor | Advanced solid tumors | DLT, MTD | NCT03993873; phase I/II; active, not recruiting |
APL-101 | c-Met inhibitor | Advanced solid tumors | ORR | NCT03175224; phase I/II; recruiting |
Ab, antibody; ORR, objective response rate; DoR, duration of response; NSCLC, non-small cell lung cancer; MTD, maximum tolerated dose; DLT, dose limiting toxicity.
Intrinsic and extrinsic mechanisms to novel fusions: a lot to be done
The identification of resistance mechanisms occurring while treating these emerging fusions is still in a purely theoretical phase, as attention is mainly focused on identifying effective drugs against these novel alterations. Therefore, the definition of resistance mechanisms can now be exclusively hypothetical, relying on the functional mechanisms behind these fusions and their associated signaling pathways and, on the other hand, on the specific targeting of the currently available drugs.
Regarding NRG1 fusions, the possibility of better understanding a potential resistance mechanism could be sought in its interaction with ERBB/HER receptors. In this case, the upregulation of other receptors like MET, HER3, and FGFRs (as demonstrated in other tumors) or the occurrence of an on-target mutation at the drug binding site, could both play a role (94). However, a case report describes the absence of novel molecular alterations at the NGS analysis performed on the rebiopsy at the progression after afatinib in a patient affected by an NRG1 fusion-positive NSCLC (95). Furthermore, in a case study involving two patients with NRG1 fusions who received treatment with afatinib, no activating mutation or copy number alteration in an ErbB receptor was observed before or after afatinib treatment (96). This suggests the potential involvement of an epigenetic event as an escape mechanism. Resistance to afatinib in ErbB receptors may follow the epigenetic pathway involving lysine methyltransferase 2D/FOXO/c-Myc, which has been reported in HER2-positive breast cancer cells developing acquired resistance to lapatinib (97).
Other examples can be borrowed from other neoplasms, where the therapeutical role of some of those fusions is already established. For example, FGFR inhibitors are nowadays a reality in cholangiocarcinoma treatment. A retrospective analysis of 17 patients investigating resistance mechanisms to these treatments revealed the emergence of novel FGFR2 mutations in 64.7% and alterations in MAPK pathway genes in 52.9% of patients, with 41.2% developing alterations in both the FGFR2 and MAPK pathways (98). Facchinetti et al. evaluated 56 patients with FGFR-driven urothelial cancer who developed resistance to FGFR inhibitors. An NGS analysis at progression identified single mutations in the FGFR tyrosine kinase domain in 33% of cases and alterations in the PI3K-mTOR pathway in 52% of patients (99).
Discussion
The landscape of treating gene fusions in NSCLC is currently witnessing significant advancements. Nonetheless, these important developments introduce novel challenges, particularly related to disease progression. The wide knowledge accumulated over time regarding the older driver ROS1 has paved the way for a more comprehensive understanding of resistance mechanisms associated with the selective targeting of this gene fusion. As a result, several data are nowadays available to overcome resistance in ROS1-targeted therapies. Conversely, resistance mechanisms to selective therapies targeting RET and NTRK gene fusions remain rather enigmatic. The knowledge gap on resistance mechanisms to RET and NTRK inhibitors may be related to the relatively recent availability of selective TKIs (such as selpercatinib, pralsetinib, repotrectinib, and entrectinib). The limited time since their introduction, as well as the prolonged benefit they guarantee, hamper the collection of a sufficient amount of clinical data, making it challenging for clinicians to confidently choose a therapeutic approach after disease progression. This highlights the urgent need for deepening our understanding of resistance mechanisms in patients with NSCLC harboring these gene fusions.
It is therefore crucial to collect real-world data that highlight and study the characteristics of these patients. In this light, an international multicenter study, involving 218 patients, the RET-MAP study, has sought to provide real-world data into the treatment of advanced NSCLC with RET fusions (100).
Performing a re-biopsy at the time of disease progression may constitute a critical step in managing oncogene-addicted NSCLC. This approach contributes to a more comprehensive understanding of disease evolution and may guide therapeutic decisions (101). Even in cases of oligoprogression, conducting a new biopsy at the site of progression can provide valuable insights for therapy management, microenvironment and molecular characteristics (5). By analyzing the tissue from a novel biopsy, clinicians can identify emerging alterations and determine whether they are contributing to treatment resistance.
This information is invaluable in some patients for tailoring the therapeutic approach and selecting the most appropriate treatment strategy (101). In addition to tissue samples, liquid biopsy has progressively gained importance, particularly in this setting. Analyzing circulating tumor DNA (ctDNA) or RNA (ctRNA) provides a comprehensive evaluation of the genetic landscape of the disease, unveiling possible resistance mechanisms (102). Although recent studies have shown promising outcomes, identifying gene fusions from ctRNA still presents a series of issues to face. In the context of acquired resistance in oncogene-driven NSCLC, adopting a “plasma first” strategy is now deemed suitable, given its potential to overcome the well-know limitations associated with tissue sampling (102,103).
About disease progression management, the adoption of combinatorial strategies involving multiple targeted drugs may represent a promising therapeutic approach. Given the relatively high incidence of MET amplification as a common resistance mechanism across different oncogene-addicted NSCLC subtypes, exploring potential combinations with MET inhibitors, such as cabozantinib, crizotinib, or capmatinib, is of interest. For instance, Rosen et al. demonstrated that the combination of selpercatinib with crizotinib could be effective in patients progressing due to MET amplification (81). Additionally, a promising response was reported in novel ISOC1-RET fusion lung cancer patient with the combination of selpercatinib and capmatinib. The authors observed a durable complete response to selpercatinib, and when resistance emerged through MET amplification, it was successfully overcome by the combined use of selpercatinib and capmatinib (104). The combination of TKIs to overcome secondary resistance due to MET amplification has already been investigated in EGFR-mutant NSCLC experiencing progression after osimertinib. In these patients, the combination of osimertinib and savolitinib demonstrated satisfactory efficacy with acceptable toxicity levels (105).
However, while this therapeutic approach may yield promising results regarding disease control and extended survival, it is crucial to exercise prudence and monitor potential adverse effects. Unfortunately, in the context of non-ALK gene fusions, data remains insufficient, and further studies are necessary to establish concrete evidence regarding the safety and efficacy of the combination of TKI therapy.
Conclusions
In this review, we provided an overview of the state-of-the-art of NSCLC fusions (excluding ALK), the available treatments, and, especially, the related resistance mechanisms. Moreover, we highlighted the treatment landscape of rare fusions, such as NRG1, FGFR, and MET, which reveals many unmet needs starting from the lack of therapies supported by strong clinical data and, consequently, the absence of evidence about potential resistance mechanisms. Clinical data about combinatorial strategies to overcome resistance mechanisms are needed. Non-genetic resistance mechanisms, such as microenvironmental changes in growth factor concentration and inefficient blood-barrier penetration of some drugs, should also be considered.
Acknowledgments
Funding: This work was supported by
Footnote
Provenance and Peer Review: This article was commissioned by the Guest Editors (Fabrizio Tabbò, Umberto Malapelle, Maria Lucia Reale and Angela Listì) for the series “How to Detect and Treat NSCLC Patients with Oncogenic Fusions” published in Precision Cancer Medicine. The article has undergone external peer review.
Peer Review File: Available at https://pcm.amegroups.com/article/view/10.21037/pcm-24-8/prf
Conflicts of Interest: All authors have completed the ICMJE uniform disclosure form (available at https://pcm.amegroups.com/article/view/10.21037/pcm-24-8/coif). The series “How to Detect and Treat NSCLC Patients with Oncogenic Fusions” was commissioned by the editorial office without any funding or sponsorship. S.P. reports payment or honoraria for lectures, presentations, speakers bureaus, etc. from Astra-Zeneca, Eli-Lilly, BMS, MSD, Takeda, Amgen, Novartis, and Roche, and reports participation on a Data Safety Monitoring Board or Advisory Board of Merck-Sharp & Dome, Pfizer, Novartis, Bristol-Myers Squibb, Astra-Zeneca, and Roche. L.B. reports payment or honoraria for lectures, presentations, speakers bureaus, etc. from Astra-Zeneca, MSD, Roche, and Takeda, as well as support for attending meetings and/or travel from Takeda and Sanofi. The authors have no other conflicts of interest to declare.
Ethical Statement: The authors are accountable for all aspects of the work in ensuring that questions related to the accuracy or integrity of any part of the work are appropriately investigated and resolved.
Open Access Statement: This is an Open Access article distributed in accordance with the Creative Commons Attribution-NonCommercial-NoDerivs 4.0 International License (CC BY-NC-ND 4.0), which permits the non-commercial replication and distribution of the article with the strict proviso that no changes or edits are made and the original work is properly cited (including links to both the formal publication through the relevant DOI and the license). See: https://creativecommons.org/licenses/by-nc-nd/4.0/.
References
- Wang M, Herbst RS, Boshoff C. Toward personalized treatment approaches for non-small-cell lung cancer. Nat Med 2021;27:1345-56. [Crossref] [PubMed]
- Rebuzzi SE, Zullo L, Rossi G, et al. Novel Emerging Molecular Targets in Non-Small Cell Lung Cancer. Int J Mol Sci 2021;22:2625. [Crossref] [PubMed]
- Sattler M, Griffin JD. Molecular mechanisms of transformation by the BCR-ABL oncogene. Semin Hematol 2003;40:4-10. [Crossref] [PubMed]
- Tuna M, Amos CI, Mills GB. Molecular mechanisms and pathobiology of oncogenic fusion transcripts in epithelial tumors. Oncotarget 2019;10:2095-111. [Crossref] [PubMed]
- Pilotto S, Molina-Vila MA, Karachaliou N, et al. Integrating the molecular background of targeted therapy and immunotherapy in lung cancer: a way to explore the impact of mutational landscape on tumor immunogenicity. Transl Lung Cancer Res 2015;4:721-7. [PubMed]
- Choudhury NJ, Lavery JA, Brown S, et al. The GENIE BPC NSCLC Cohort: A Real-World Repository Integrating Standardized Clinical and Genomic Data for 1,846 Patients with Non-Small Cell Lung Cancer. Clin Cancer Res 2023;29:3418-28. [Crossref] [PubMed]
- Li B, Qu H, Zhang J, et al. Genomic characterization and outcome evaluation of kinome fusions in lung cancer revealed novel druggable fusions. NPJ Precis Oncol 2021;5:81. [Crossref] [PubMed]
- Luca C, Pepe F, Pisapia P, et al. RNA-based next-generation sequencing in non-small-cell lung cancer in a routine setting: an experience from an Italian referral center. Per Med 2022;19:395-401. [Crossref] [PubMed]
- Chen J, Xu C, Lv J, et al. Clinical characteristics and targeted therapy of different gene fusions in non-small cell lung cancer: a narrative review. Transl Lung Cancer Res 2023;12:895-908. [Crossref] [PubMed]
- Bergethon K, Shaw AT, Ou SH, et al. ROS1 rearrangements define a unique molecular class of lung cancers. J Clin Oncol 2012;30:863-70. [Crossref] [PubMed]
- Drilon A, Jenkins C, Iyer S, et al. ROS1-dependent cancers - biology, diagnostics and therapeutics. Nat Rev Clin Oncol 2021;18:35-55. [Crossref] [PubMed]
- Shaw AT, Riely GJ, Bang YJ, et al. Crizotinib in ROS1-rearranged advanced non-small-cell lung cancer (NSCLC): updated results, including overall survival, from PROFILE 1001. Ann Oncol 2019;30:1121-6. [Crossref] [PubMed]
- Landi L, Chiari R, Tiseo M, et al. Crizotinib in MET-Deregulated or ROS1-Rearranged Pretreated Non-Small Cell Lung Cancer (METROS): A Phase II, Prospective, Multicenter, Two-Arms Trial. Clin Cancer Res 2019;25:7312-9. [Crossref] [PubMed]
- Michels S, Massutí B, Schildhaus HU, et al. Safety and Efficacy of Crizotinib in Patients With Advanced or Metastatic ROS1-Rearranged Lung Cancer (EUCROSS): A European Phase II Clinical Trial. J Thorac Oncol 2019;14:1266-76. [Crossref] [PubMed]
- Stanzione B, Del Conte A, Bertoli E, et al. Therapeutical Options in ROS1-Rearranged Advanced Non Small Cell Lung Cancer. Int J Mol Sci 2023;24:11495. [Crossref] [PubMed]
- Drilon A, Chiu CH, Fan Y, et al. Long-Term Efficacy and Safety of Entrectinib in ROS1 Fusion-Positive NSCLC. JTO Clin Res Rep 2022;3:100332. [Crossref] [PubMed]
- Hendriks LE, Kerr KM, Menis J, et al. Oncogene-addicted metastatic non-small-cell lung cancer: ESMO Clinical Practice Guideline for diagnosis, treatment and follow-up. Ann Oncol 2023;34:339-57. [Crossref] [PubMed]
- Lim SM, Kim HR, Lee JS, et al. Open-Label, Multicenter, Phase II Study of Ceritinib in Patients With Non-Small-Cell Lung Cancer Harboring ROS1 Rearrangement. J Clin Oncol 2017;35:2613-8. [Crossref] [PubMed]
- Shaw AT, Solomon BJ, Chiari R, et al. Lorlatinib in advanced ROS1-positive non-small-cell lung cancer: a multicentre, open-label, single-arm, phase 1-2 trial. Lancet Oncol 2019;20:1691-701. [Crossref] [PubMed]
- Drilon A, Camidge DR, Lin JJ, et al. Repotrectinib in ROS1 Fusion-Positive Non-Small-Cell Lung Cancer. N Engl J Med 2024;390:118-31. [Crossref] [PubMed]
- Pérol M, Yang N, Choi CM, et al. Efficacy and safety of taletrectinib in patients (Pts) with ROS1+ non-small cell lung cancer (NSCLC): Interim analysis of global TRUST-II study. Ann Oncol 2023;34:S788-9. [Crossref]
- Facchinetti F, Rossi G, Bria E, et al. Oncogene addiction in non-small cell lung cancer: Focus on ROS1 inhibition. Cancer Treat Rev 2017;55:83-95. [Crossref] [PubMed]
- Roys A, Chang X, Liu Y, et al. Resistance mechanisms and potent-targeted therapies of ROS1-positive lung cancer. Cancer Chemother Pharmacol 2019;84:679-88. [Crossref] [PubMed]
- Gou W, Zhou X, Liu Z, et al. CD74-ROS1 G2032R mutation transcriptionally up-regulates Twist1 in non-small cell lung cancer cells leading to increased migration, invasion, and resistance to crizotinib. Cancer Lett 2018;422:19-28. [Crossref] [PubMed]
- Yang MH, Wu KJ. TWIST activation by hypoxia inducible factor-1 (HIF-1): implications in metastasis and development. Cell Cycle 2008;7:2090-6. [Crossref] [PubMed]
- Gainor JF, Tseng D, Yoda S, et al. Patterns of Metastatic Spread and Mechanisms of Resistance to Crizotinib in ROS1-Positive Non-Small-Cell Lung Cancer. JCO Precis Oncol 2017;2017:PO.17.00063.
- Patil T, Smith DE, Bunn PA, et al. The Incidence of Brain Metastases in Stage IV ROS1-Rearranged Non-Small Cell Lung Cancer and Rate of Central Nervous System Progression on Crizotinib. J Thorac Oncol 2018;13:1717-26. [Crossref] [PubMed]
- Lin JJ, Choudhury NJ, Yoda S, et al. Spectrum of Mechanisms of Resistance to Crizotinib and Lorlatinib in ROS1 Fusion-Positive Lung Cancer. Clin Cancer Res 2021;27:2899-909. [Crossref] [PubMed]
- Lin JJ, Johnson T, Lennerz JK, et al. Resistance to lorlatinib in ROS1 fusion-positive non-small cell lung cancer. J Clin Oncol 2020;38:9611. [Crossref]
- McCoach CE, Le AT, Gowan K, et al. Resistance Mechanisms to Targeted Therapies in ROS1(+) and ALK(+) Non-small Cell Lung Cancer. Clin Cancer Res 2018;24:3334-47. [Crossref] [PubMed]
- Facchinetti F, Loriot Y, Kuo MS, et al. Crizotinib-Resistant ROS1 Mutations Reveal a Predictive Kinase Inhibitor Sensitivity Model for ROS1- and ALK-Rearranged Lung Cancers. Clin Cancer Res 2016;22:5983-91. [Crossref] [PubMed]
- Watanabe J, Furuya N, Fujiwara Y. Appearance of a BRAF Mutation Conferring Resistance to Crizotinib in Non-Small Cell Lung Cancer Harboring Oncogenic ROS1 Fusion. J Thorac Oncol 2018;13:e66-9. [Crossref] [PubMed]
- Li D, Liu J, Zhang X, et al. Combined Lorlatinib, Dabrafenib, and Trametinib Treatment for ROS1-Rearranged Advanced Non-Small-Cell Lung Cancer with a Lorlatinib-Induced BRAF V600E Mutation: A Case Report. Cancer Manag Res 2022;14:3175-9. [Crossref] [PubMed]
- Zhu YC, Lin XP, Li XF, et al. Concurrent ROS1 gene rearrangement and KRAS mutation in lung adenocarcinoma: A case report and literature review. Thorac Cancer 2018;9:159-63. [Crossref] [PubMed]
- Sato H, Schoenfeld AJ, Siau E, et al. MAPK Pathway Alterations Correlate with Poor Survival and Drive Resistance to Therapy in Patients with Lung Cancers Driven by ROS1 Fusions. Clin Cancer Res 2020;26:2932-45. [Crossref] [PubMed]
- Davies KD, Mahale S, Astling DP, et al. Resistance to ROS1 inhibition mediated by EGFR pathway activation in non-small cell lung cancer. PLoS One 2013;8:e82236. [Crossref] [PubMed]
- Dziadziuszko R, Le AT, Wrona A, et al. An Activating KIT Mutation Induces Crizotinib Resistance in ROS1-Positive Lung Cancer. J Thorac Oncol 2016;11:1273-81. [Crossref] [PubMed]
- Tyler LC, Le AT, Chen N, et al. MET gene amplification is a mechanism of resistance to entrectinib in ROS1+ NSCLC. Thorac Cancer 2022;13:3032-41. [Crossref] [PubMed]
- Valent A, Danglot G, Bernheim A. Mapping of the tyrosine kinase receptors trkA (NTRK1), trkB (NTRK2) and trkC(NTRK3) to human chromosomes 1q22, 9q22 and 15q25 by fluorescence in situ hybridization. Eur J Hum Genet 1997;5:102-4. [Crossref] [PubMed]
- Lamballe F, Klein R, Barbacid M. trkC, a new member of the trk family of tyrosine protein kinases, is a receptor for neurotrophin-3. Cell 1991;66:967-79. [Crossref] [PubMed]
- Amatu A, Sartore-Bianchi A, Bencardino K, et al. Tropomyosin receptor kinase (TRK) biology and the role of NTRK gene fusions in cancer. Ann Oncol 2019;30:viii5-viii15.
- Overbeck TR, Reiffert A, Schmitz K, et al. NTRK Gene Fusions in Non-Small-Cell Lung Cancer: Real-World Screening Data of 1068 Unselected Patients. Cancers (Basel) 2023;15:2966. [Crossref] [PubMed]
- Vaishnavi A, Capelletti M, Le AT, et al. Oncogenic and drug-sensitive NTRK1 rearrangements in lung cancer. Nat Med 2013;19:1469-72. [Crossref] [PubMed]
- Xia H, Xue X, Ding H, et al. Evidence of NTRK1 Fusion as Resistance Mechanism to EGFR TKI in EGFR+ NSCLC: Results From a Large-Scale Survey of NTRK1 Fusions in Chinese Patients With Lung Cancer. Clin Lung Cancer 2020;21:247-54. [Crossref] [PubMed]
- Farago AF, Taylor MS, Doebele RC, et al. Clinicopathologic Features of Non-Small-Cell Lung Cancer Harboring an NTRK Gene Fusion. JCO Precis Oncol 2018;2018:PO.18.00037.
- Lamberti G, Andrini E, Sisi M, et al. Beyond EGFR, ALK and ROS1: Current evidence and future perspectives on newly targetable oncogenic drivers in lung adenocarcinoma. Crit Rev Oncol Hematol 2020;156:103119. [Crossref] [PubMed]
- Lin JJ, Tan DSW, Kummar S, et al. Long-term efficacy and safety of larotrectinib in patients with tropomyosin receptor kinase (TRK) fusion lung cancer. J Clin Oncol 2023;41:9056. [Crossref]
- Cho BC, Chiu CH, Massarelli E, et al. Updated efficacy and safety of entrectinib in patients (pts) with locally advanced/metastatic NTRK fusion-positive (fp) non-small cell lung cancer (NSCLC). J Clin Oncol 2023;41:9047. [Crossref]
- Liu F, Wei Y, Zhang H, et al. NTRK Fusion in Non-Small Cell Lung Cancer: Diagnosis, Therapy, and TRK Inhibitor Resistance. Front Oncol 2022;12:864666. [Crossref] [PubMed]
- Russo M, Misale S, Wei G, et al. Acquired Resistance to the TRK Inhibitor Entrectinib in Colorectal Cancer. Cancer Discov 2016;6:36-44. [Crossref] [PubMed]
- Hong DS, Bauer TM, Lee JJ, et al. Larotrectinib in adult patients with solid tumours: a multi-centre, open-label, phase I dose-escalation study. Ann Oncol 2019;30:325-31. [Crossref] [PubMed]
- Drilon A, Li G, Dogan S, et al. What hides behind the MASC: clinical response and acquired resistance to entrectinib after ETV6-NTRK3 identification in a mammary analogue secretory carcinoma (MASC). Ann Oncol 2016;27:920-6. [Crossref] [PubMed]
- Drilon A, Laetsch TW, Kummar S, et al. Efficacy of Larotrectinib in TRK Fusion-Positive Cancers in Adults and Children. N Engl J Med 2018;378:731-9. [Crossref] [PubMed]
- Hyman D, Kummar S, Farago A, et al. Abstract CT127: Phase I and expanded access experience of LOXO-195 (BAY 2731954), a selective next-generation TRK inhibitor (TRKi). Cancer Res 2019;79:CT127. -CT. [Crossref]
- Drilon A, Ou SI, Cho BC, et al. Repotrectinib (TPX-0005) Is a Next-Generation ROS1/TRK/ALK Inhibitor That Potently Inhibits ROS1/TRK/ALK Solvent- Front Mutations. Cancer Discov 2018;8:1227-36. [Crossref] [PubMed]
- Solomon BJ, Drilon A, Lin JJ, et al. 1372P Repotrectinib in patients (pts) with NTRK fusion-positive (NTRK+) advanced solid tumors, including NSCLC: Update from the phase I/II TRIDENT-1 trial. Ann Oncol 2023;34:S787-8. [Crossref]
- Somwar R, Hofmann NE, Smith B, et al. NTRK kinase domain mutations in cancer variably impact sensitivity to type I and type II inhibitors. Commun Biol 2020;3:776. [Crossref] [PubMed]
- Katayama R, Gong B, Togashi N, et al. The new-generation selective ROS1/NTRK inhibitor DS-6051b overcomes crizotinib resistant ROS1-G2032R mutation in preclinical models. Nat Commun 2019;10:3604. [Crossref] [PubMed]
- Cocco E, Lee JE, Kannan S, et al. TRK xDFG Mutations Trigger a Sensitivity Switch from Type I to II Kinase Inhibitors. Cancer Discov 2021;11:126-41. [Crossref] [PubMed]
- Vaishnavi A, Scherzer MT, Kinsey CG, et al. Inhibition of MEK1/2 Forestalls the Onset of Acquired Resistance to Entrectinib in Multiple Models of NTRK1-Driven Cancer. Cell Rep 2020;32:107994. [Crossref] [PubMed]
- Fuse MJ, Okada K, Oh-Hara T, et al. Mechanisms of Resistance to NTRK Inhibitors and Therapeutic Strategies in NTRK1-Rearranged Cancers. Mol Cancer Ther 2017;16:2130-43. [Crossref] [PubMed]
- Takumi Y, Arai S, Suzuki C, et al. MET kinase inhibitor reverses resistance to entrectinib induced by hepatocyte growth factor in tumors with NTRK1 or ROS1 rearrangements. Cancer Med 2023;12:5809-20. [Crossref] [PubMed]
- Ishizaka Y, Itoh F, Tahira T, et al. Human ret proto-oncogene mapped to chromosome 10q11.2. Oncogene 1989;4:1519-21. [PubMed]
- Ferrara R, Auger N, Auclin E, et al. Clinical and Translational Implications of RET Rearrangements in Non-Small Cell Lung Cancer. J Thorac Oncol 2018;13:27-45. [Crossref] [PubMed]
- Drilon A, Subbiah V, Gautschi O, et al. Selpercatinib in Patients With RET Fusion-Positive Non-Small-Cell Lung Cancer: Updated Safety and Efficacy From the Registrational LIBRETTO-001 Phase I/II Trial. J Clin Oncol 2023;41:385-94. [Crossref] [PubMed]
- Griesinger F, Curigliano G, Thomas M, et al. Safety and efficacy of pralsetinib in RET fusion-positive non-small-cell lung cancer including as first-line therapy: update from the ARROW trial. Ann Oncol 2022;33:1168-78. [Crossref] [PubMed]
- Zhou C, Solomon B, Loong HH, et al. First-Line Selpercatinib or Chemotherapy and Pembrolizumab in RET Fusion-Positive NSCLC. N Engl J Med 2023;389:1839-50. [Crossref] [PubMed]
- Cooper AJ, Drilon AE, Rotow JK, et al. First results from the RETgistry: A global consortium for the study of resistance to RET inhibition in RET-altered solid tumors. J Clin Oncol 2023;41:9065. [Crossref]
- Gainor J, Curigliano G, Doebele RC, et al. OA05.02 Analysis of Resistance Mmechanisms to Pralsetinib in Patients with RET Fusion-Positive Non-Small Cell Lung Cancer (NSCLC) from the ARROW Study. J Thorac Oncol 2021;16:S5. [Crossref]
- Miyazaki I, Odintsov I, Ishida K, et al. Vepafestinib is a pharmacologically advanced RET-selective inhibitor with high CNS penetration and inhibitory activity against RET solvent front mutations. Nat Cancer 2023;4:1345-61. [Crossref] [PubMed]
- Drilon A, Rogers E, Zhai D, et al. 506P - TPX-0046 is a novel and potent RET/SRC inhibitor for RET-driven cancers. Ann Oncol 2019;30:v190-1. [Crossref]
- Zhou Q, Wu YL, Zheng X, et al. A phase I study of KL590586, a next-generation selective RET inhibitor, in patients with RET-altered solid tumors. J Clin Oncol 2023;41:3007. [Crossref]
- Subbiah V, Zhong J, Lu Y, et al. The development of APS03118, a potent next-generation RET inhibitor for treating RET-inhibitor-resistant patients. J Clin Oncol 2022;40:e15107. [Crossref]
- Novello S, Califano R, Reinmuth N, et al. RET Fusion-Positive Non-small Cell Lung Cancer: The Evolving Treatment Landscape. Oncologist 2023;28:402-13. [Crossref] [PubMed]
- Lin JJ, Liu SV, McCoach CE, et al. Mechanisms of resistance to selective RET tyrosine kinase inhibitors in RET fusion-positive non-small-cell lung cancer. Ann Oncol 2020;31:1725-33. [Crossref] [PubMed]
- Subbiah V, Shen T, Terzyan SS, et al. Structural basis of acquired resistance to selpercatinib and pralsetinib mediated by non-gatekeeper RET mutations. Ann Oncol 2021;32:261-8. [Crossref] [PubMed]
- Solomon BJ, Tan L, Lin JJ, et al. RET Solvent Front Mutations Mediate Acquired Resistance to Selective RET Inhibition in RET-Driven Malignancies. J Thorac Oncol 2020;15:541-9. [Crossref] [PubMed]
- Gainor JF, Curigliano G, Kim DW, et al. Pralsetinib for RET fusion-positive non-small-cell lung cancer (ARROW): a multi-cohort, open-label, phase 1/2 study. Lancet Oncol 2021;22:959-69. [Crossref] [PubMed]
- Subbiah V, Shen T, Tetzlaff M, et al. Patient-driven discovery and post-clinical validation of NTRK3 fusion as an acquired resistance mechanism to selpercatinib in RET fusion-positive lung cancer. Ann Oncol 2021;32:817-9. [Crossref] [PubMed]
- Marinello A, Vasseur D, Conci N, et al. 1007P Mechanisms of primary and secondary resistance to RET inhibitors in patients with RET-positive advanced NSCLC. Ann Oncol 2022;33:S1013-4. [Crossref]
- Rosen EY, Johnson ML, Clifford SE, et al. Overcoming MET-Dependent Resistance to Selective RET Inhibition in Patients with RET Fusion-Positive Lung Cancer by Combining Selpercatinib with Crizotinib. Clin Cancer Res 2021;27:34-42. [Crossref] [PubMed]
- Fernandez-Cuesta L, Plenker D, Osada H, et al. CD74-NRG1 fusions in lung adenocarcinoma. Cancer Discov 2014;4:415-22. [Crossref] [PubMed]
- Drilon A, Duruisseaux M, Han JY, et al. Clinicopathologic Features and Response to Therapy of NRG1 Fusion-Driven Lung Cancers: The eNRGy1 Global Multicenter Registry. J Clin Oncol 2021;39:2791-802. [Crossref] [PubMed]
- Rosas D, Raez LE, Russo A, et al. Neuregulin 1 Gene (NRG1). A Potentially New Targetable Alteration for the Treatment of Lung Cancer. Cancers (Basel) 2021;13:5038. [Crossref] [PubMed]
- Schram AM, O'Reilly EM, O'Kane GM, et al. Efficacy and safety of zenocutuzumab in advanced pancreas cancer and other solid tumors harboring NRG1 fusions. J Clin Oncol 2021;39:3003. [Crossref]
- Ornitz DM, Itoh N. The Fibroblast Growth Factor signaling pathway. Wiley Interdiscip Rev Dev Biol 2015;4:215-66. [Crossref] [PubMed]
- Wu YM, Su F, Kalyana-Sundaram S, et al. Identification of targetable FGFR gene fusions in diverse cancers. Cancer Discov 2013;3:636-47. [Crossref] [PubMed]
- Lim SH, Sun JM, Choi YL, et al. Efficacy and safety of dovitinib in pretreated patients with advanced squamous non-small cell lung cancer with FGFR1 amplification: A single-arm, phase 2 study. Cancer 2016;122:3024-31. [Crossref] [PubMed]
- Pham C, Lang D, Iams WT. Successful Treatment and Retreatment With Erdafitinib for a Patient With FGFR3-TACC3 Fusion Squamous NSCLC: A Case Report. JTO Clin Res Rep 2023;4:100511. [Crossref] [PubMed]
- Davies KD, Ng TL, Estrada-Bernal A, et al. Dramatic Response to Crizotinib in a Patient with Lung Cancer Positive for an HLA-DRB1-MET Gene Fusion. JCO Precis Oncol 2017;2017:PO.17.00117.
- Zhu YC, Wang WX, Xu CW, et al. Identification of a novel crizotinib-sensitive MET-ATXN7L1 gene fusion variant in lung adenocarcinoma by next generation sequencing. Ann Oncol 2018;29:2392-3. [Crossref] [PubMed]
- Riedel R, Fassunke J, Scheel AH, et al. MET Fusions in NSCLC: Clinicopathologic Features and Response to MET Inhibition. J Thorac Oncol 2024;19:160-5. [Crossref] [PubMed]
- Xu C, Wang WX, Chen Y, et al. Prevalence of EGFR gene fusions in a large cohort of Chinese patients with non-small cell lung cancer (NSCLC). J Clin Oncol 2018;36:e13538. [Crossref]
- Blangé D, Stroes CI, Derks S, et al. Resistance mechanisms to HER2-targeted therapy in gastroesophageal adenocarcinoma: A systematic review. Cancer Treat Rev 2022;108:102418. [Crossref] [PubMed]
- Wu X, Zhang D, Shi M, et al. Successful targeting of the NRG1 fusion reveals durable response to afatinib in lung adenocarcinoma: a case report. Ann Transl Med 2021;9:1507. [Crossref] [PubMed]
- Gay ND, Wang Y, Beadling C, et al. Durable Response to Afatinib in Lung Adenocarcinoma Harboring NRG1 Gene Fusions. J Thorac Oncol 2017;12:e107-10. [Crossref] [PubMed]
- Matkar S, Sharma P, Gao S, et al. An Epigenetic Pathway Regulates Sensitivity of Breast Cancer Cells to HER2 Inhibition via FOXO/c-Myc Axis. Cancer Cell 2015;28:472-85. [Crossref] [PubMed]
- DiPeri TP, Zhao M, Evans KW, et al. Convergent MAPK pathway alterations mediate acquired resistance to FGFR inhibitors in FGFR2 fusion-positive cholangiocarcinoma. J Hepatol 2024;80:322-34. [Crossref] [PubMed]
- Facchinetti F, Hollebecque A, Braye F, et al. Resistance to Selective FGFR Inhibitors in FGFR-Driven Urothelial Cancer. Cancer Discov 2023;13:1998-2011. [Crossref] [PubMed]
- Aldea M, Marinello A, Duruisseaux M, et al. RET-MAP: An International Multicenter Study on Clinicobiologic Features and Treatment Response in Patients With Lung Cancer Harboring a RET Fusion. J Thorac Oncol 2023;18:576-86. [Crossref] [PubMed]
- Kerr KM, Bibeau F, Thunnissen E, et al. The evolving landscape of biomarker testing for non-small cell lung cancer in Europe. Lung Cancer 2021;154:161-75. [Crossref] [PubMed]
- Rolfo C, Mack P, Scagliotti GV, et al. Liquid Biopsy for Advanced NSCLC: A Consensus Statement From the International Association for the Study of Lung Cancer. J Thorac Oncol 2021;16:1647-62. [Crossref] [PubMed]
- Reclusa P, Laes JF, Malapelle U, et al. EML4-ALK translocation identification in RNA exosomal cargo (ExoALK) in NSCLC patients: a novel role for liquid biopsy. Transl Cancer Res 2019;8:S76-8. [Crossref] [PubMed]
- Leite CA, Carvalho RP, da Costa FM, et al. Selpercatinib and capmatinib combination promotes sustained complete response in novel ISOC1-RET fusion lung cancer after resistance to RET inhibitor via MET amplification Case Report. Front Oncol 2023;13:1264231. [Crossref] [PubMed]
- Sequist LV, Han JY, Ahn MJ, et al. Osimertinib plus savolitinib in patients with EGFR mutation-positive, MET-amplified, non-small-cell lung cancer after progression on EGFR tyrosine kinase inhibitors: interim results from a multicentre, open-label, phase 1b study. Lancet Oncol 2020;21:373-86. [Crossref] [PubMed]
Cite this article as: Sposito M, Scaglione IM, Dodi A, Avancini A, Trestini I, Tregnago D, Insolda J, Milella M, Pilotto S, Belluomini L. Genetic and non-genetic resistance mechanisms in non-small cell lung cancer harboring (non-ALK) oncogenic fusions. Precis Cancer Med 2024;7:1.