ALK fusions turn sixteen in lung cancer: a review on their biology, detection and therapy
Introduction
The identification of molecular events driving malignant cell fate, from initiation to aggressiveness and metastasis, fostered the development of targeted anticancer agents improving patients’ outcomes. Moving from the seminal model of chronic myeloid leukemia driven by BCR::ABL fusion, susceptible to the inhibition by imatinib and following generations of tyrosine kinase inhibitors (TKIs) (1), several targetable drivers were identified in non-small cell lung cancer (NSCLC). It is estimated that approximately 50% of non-squamous NSCLC harbor an oncogenic driver (2), translating in the possibility that half of the patients suffering from NSCLC can benefit from targeted treatments. Chronologically, after the recognition of mutations in the tyrosine kinase domain of the epidermal growth factor receptor (EGFR) as the molecular marker of sensitivity to EGFR-TKI (3,4) In NSCLC, ALK (anaplastic lymphoma kinase) rearrangements emerged as the second targetable driver and especially, as the first one as a gene fusion (5). ALK inhibition in NSCLC represents a model for precision oncology, given the impressive clinical benefit achievable in patients suffering from ALK-positive NSCLC after the introduction of specific TKI. The understanding of the biological underpinnings of ALK as an oncogenic driver and the corresponding molecular elements responsible for resistance to targeted agents are the core elements for improving outcomes in this molecularly-defined subset of patients. The evolution in the field of ALK inhibition in NSCLC can be considered a model for: (I) additional fusion-driven lung cancer (namely ROS1-positive NSCLC); (II) other ALK-dependent malignancies, where ALK alterations are pathognomonic [i.e., anaplastic large cell lymphoma (ALCL), neuroblastoma, inflammatory myofibroblastic tumor] (6); (III) diseases harboring ALK fusions in a relatively small fraction of cases, detectable with the wide utilization of molecular diagnostic techniques across histologies, suggesting a tumor “agnostic” treatment (7); (IV) generally, oncogene-driven malignancies in which the development of targeted agents and treatment strategies can retrace the one adopted for ALK-rearranged NSCLC. In this review, we aim to provide the context for which ALK fusions in lung tumors are such a model for precision oncology, approaching their biological features and addressing pragmatic aspects, namely in terms of molecular diagnostic and treatment.
Biology of ALK fusion in NSCLC
Defining ALK as a driver oncogene: historical evidence
ALK gene was firstly identified in the genetic context of a gene fusion in the pathognomonic ALCL. The recognition of a chromosomal translocation between the short arm of chromosome 2 and the long arm of chromosome 5, t(2;5)(p23;q35), led to the identification of the fusion gene involving NPM (nucleophosmin, mapped on 5q35) and ALK (2p23) (8).
The discovery and initial characterization of ALK fusions as oncogenic events in NSCLC date back to 2007. Starting from a surgical patient sample, Soda et al. identified an ALK fusion with EML4 (echinoderm microtubule-associated protein-like 4) gene (EML4::ALK) after RNA extraction, cloning procedures and retroviral infection in 3T3 fibroblasts (5). Using a phosphoproteomic approach, Rikova et al. recognized high-level of ALK phosphorylation across a wide number of lung cancer tumor specimens and cell lines, in which the presence of ALK fusions (with either EML4 or TGF as partner genes) was confirmed (9). Of interest, this latter study was the first to identify ROS1 fusions across malignancies, paving the way for the development of precision medicine in this molecular subtype as well. Soda et al. confirmed the transforming activity of ALK fusions (with both EML4 and NPM as partner genes) in 3T3 mouse fibroblasts, by in vitro and in vivo growth assays. Moreover, Ba/F3 models engineered to express EML4::ALK were able to proliferate in the absence of IL-3, suggesting the oncogenic competence of the fusion protein (5).
ALK fusions in NSCLC: from structure to function
As anticipated, the genomic events leading to ALK fusion belong to chromosomal translocations. The variety of ALK fusion partners in NSCLC is extremely rich and constantly updated (10). With regard to the most frequent fusion partner of ALK in NSCLC, EML4 maps on the short arm of chromosome 2 (2p), implying that an intrachromosomal rearrangement occur in this case (5).
While EML4 gene has different breakpoints (giving rise to different fusion variants, from v1 to v5), ALK breakpoint is conserved, located after the juxta-membrane domain and, most importantly, before the tyrosine kinase domain (Figure 1). Therefore, the fusion transcript contains the N-terminal, 5’ extremity of EML4 and the C-terminal, 3’ extremity of ALK. This general structure of the fusion transcript is maintained whichever the ALK fusion partner and the disease, in case of inter- or intra-chromosomal rearrangements. The key event is indeed represented by the conservation of a fully intact tyrosine kinase domain of ALK (Figure 1).
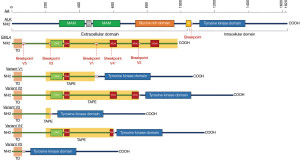
ALK fusions harbor oncogenic potential for two main reasons. The first explanation can be found in the physiological role and the expression of ALK protein in mammalian tissues. Whereas ALK seems to have a role in central and peripheral nervous system development, mRNA expression in adult human tissues is limited to the enteric innervation, brain, testis and placenta. ALK protein is detected in brain neurons, pericytes and endothelial cells (11,12). ALK expression across the body is indeed regulated by a negative promoter. The chromosomic rearrangements generating gene fusions release the tyrosine kinase domain of ALK from this transcriptional control. The second reason relies on the activation of ALK signaling in the presence of receptor dimerization. Through its coiled-coil domain, EML4 sustains dimerization of the EML4::ALK fusion protein, leading to constitutive intracellular signaling (5). This latter is mediated by molecular pathways such as MAPK, PI3K/mTOR, JAK/STAT, PLCɣ, relying on the cascade phosphorylation of effectors and mediators towards the cellular nucleus, where transcription factors implied in transformation are recruited, starting and maintaining a malignant phenotype (13).
Molecular diagnostics for the detection of ALK fusions in NSCLC
Since the original report by Soda et al. in 2007 (5), several studies have investigated the presence of ALK rearrangements in NSCLC with different techniques (14-16). ALK fusions mainly occur in adenocarcinomas showing a solid, acinar or cribriform pattern, often accompanied by the finding of a proportion of “signet ring” cells with intracytoplasmic mucus production (17-19). ALK-rearranged adenocarcinomas generally express TTF1 and napsin A and are negative for p63/p40 (17,19). Nevertheless, none of these morphologic or immunohistochemical features are per se indicative of the presence of ALK rearrangement, and even different histologies of NSCLC, including squamous cell carcinoma, should be tested for ALK fusions when dealing with light/never smokers or young patients, particularly in small biopsies when an adenocarcinoma component could be missed (20). At the molecular level, although ALK rearrangements are regarded as essentially mutually exclusive with genetic alterations in other oncogenic drivers (21), reports demonstrated the occurrence of concomitant ALK rearrangements with EGFR, KRAS or other druggable or non-druggable oncogenic drivers (22-24).
Approval for the use of ALK inhibitors in routine management of patients with ALK-positive NSCLC by the Food and Drug Administration (FDA) and the European Medicines Agency (EMA) is regulated by the preliminary detection of ALK gene fusion by using fluorescence in situ hybridization (FISH) assay, immunohistochemistry (IHC), extractive technologies or integrated approaches (Table 1) (14,16).
Table 1
Methods | Sensibility | Specificity | Advantages | Limitations | Tumor sampling | Principal setting |
---|---|---|---|---|---|---|
IHC | >95% | >95% | The only IHC test with direct access to TKI in case of positivity; rapid TAT; limited amount of tissue; each batch in automated immunostainer; standardized protocol; technique available worldwide in routine practice; easy and objective interpretation by pathologists | Single test; surrogate detection method (heterogeneous partner gene-dependent performance); be aware on primary Ab clone used (ALK D5F3 companion diagnostic is the most performant); does not recognize gene fusion partner or variant | FFPE tissue (including cell block) and cytology | ALK rearrangement screening tool; orthogonal technique flanking NGS or other methods in indeterminate/doubtful results |
FISH | 85–90% | 95% | In situ assay; correspondence with morphology; utilization of smeared cytological samples, not suitable for IHC; better understanding of the biology behind the rearrangement (deletion/insertion/inversion); quantitative evaluation of the percentage of rearranged cells | Expensive method; false positives/false negatives; long TAT (workflow: 2 days); require expertise (subjective interpretation); single gene assay; do not recognize gene fusion partner or variant | FFPE tissue (including cell block) and cytology | Orthogonal technique flanking NGS or other methods in indeterminate/doubtful results |
RT-PCR and multiplex RT-PCR | 92.4% (FFPE) | 97.8% (FFPE) | Simple and relatively inexpensive method | Variable yield in RNA extraction from FFPE samples; detect only known and common fusion partner, miss rare variants (only imbalance expression detected) | FFPE tissue (including cell block) and cytology; plasma | |
Rapid TAT (workflow: 2–3 h + extraction time) | ||||||
NGS | 100% | 97.7% | Require small RNA amount; qualitative and quantitative assay; detect known and novel fusion partner, complex fusions and rare variants, multiplex assay; dual detection strategy (imbalance expression and specific fusion partner) | Variable yield in RNA extraction from FFPE samples; laborious method; long TAT (workflow: 3–4 days + extraction time) require expertise | FFPE tissue (including cell block) and cytology; plasma DNA | |
IdyllaTM* | 97% | 100% | User friendly; direct FFPE tissue input (does not require RNA extraction); minimal tissue requirement; rapid TAT (workflow: 3 h); multiplex assay | Detect only known and common fusion partner, miss rare variants | FFPE tissue (including cell block) and cytology | |
NanoString* | 97% | 99% | Multiplex assay testing; dual detection strategy (imbalance expression and specific fusion partner) | Technologies not fully implemented in the clinical setting; require expertise; high cost | FFPE tissue |
*, clinical utility and performance of an ultrarapid multiplex RNA-based assay for detection of ALK, ROS1, RET, and NTRK1/2/3 rearrangements and MET Exon 14 skipping alterations. NSCLC, non-small cell lung cancer; IHC, immunohistochemistry; TKI, tyrosine kinase inhibitor; TAT, tourn-around-time; FFPE, formalin-fixed paraffin-embedded; NGS, next-generation sequencing; FISH, fluorescence in situ hybridization; RT-PCR, reverse transcriptase polymerase chain reaction.
FISH
In brief, FISH has been initially considered the gold standard technique in the first clinical trials with crizotinib (14-16,25), but this method is technically challenging, somehow difficult to interpret, then requiring specific expertise, and relatively expensive. ALK Break Apart FISH Probe Kit was used to detect ALK breakage and a minimum of 50 non-overlapping cancer cell nuclei should be examined for each case. The interpretation of FISH signals is performed according to the criteria suggested by the international guidelines (14-16,25), and the rearrangement-positive cells were defined as those with split signals or isolated red (3’) signals with a frequency ≥15% of tumor cells (Figures 2,3).
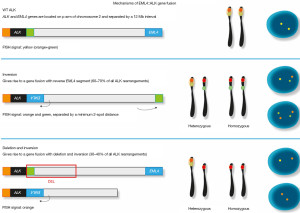
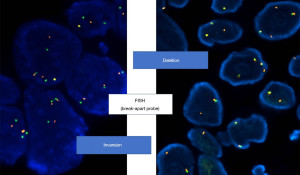
IHC
Evidence of the role of IHC in detection of ALK protein expression and its advantages over FISH in terms of availability and costs subsequently emerged (14,26-29). Among various primary antibodies clones, D5F3 showed the best performance, particularly when used inside the Ventana ALK (D5F3) companion diagnostic (CDx) (Figure 4) (27-31). Of note, several experiences have underlined the superiority of IHC over FISH in predicting ALK positivity and efficacy of ALK inhibitors (32-34).
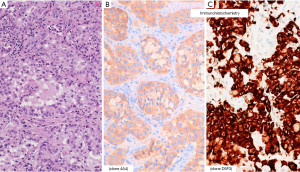
In the recent past, several algorithms incorporating the coordinated use of FISH and IHC have been developed aiming at the correct identification of ALK gene fusion in every single patient potentially candidate to ALK inhibitors (Figure 5).
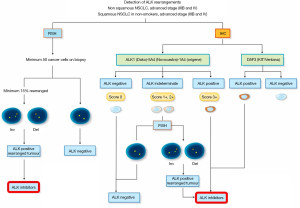
Reverse transcriptase polymerase chain reaction (RT-PCR)
Among extractive molecular technologies, RT-PCR is a reliable, sensitive and specific method to detect ALK gene rearrangements. On the other hand, this technique requires some skills and availability of good quality RNA, since tumor tissue generally derive from formalin-fixed, paraffin-embedded (FFPE) samples (30). In addition, uncommon variants of ALK gene fusion may be uncovered. A meta-analysis on 18 articles including 21 studies and involving 2,800 samples from NSCLC patients showed an overall pooled sensitivity of 92.4% and specificity of 97.8% (35).
Nano String assay is a technology that detects known fusion gene transcripts on FFPE tissue by combination of 3’ overexpression and fusion-specific detection probes (36). This technology acts as a multiplexed mRNA-based assay and shows a great concordance rate when compared with IHC (98%) and FISH (87.5–100%) for ALK gene fusion, with a specificity of 98.8%. Nevertheless, the assay requires a high content of RNA (100–200 nanograms) and data on cytology samples have not been reported so far (36,37).
More recently, IdyllaTM GeneFusion assay (a rapid and fully-automated platform simultaneously detecting ALK, ROS1, RET and NTRK1/2/3 and MET exon 14 skipping mutations) showed a 100% sensitivity in detecting fusions of ALK, ROS1, RET, NTRK1, and MET exon 14 skipping and 83% sensitivity for NTRK2/3 fusions, when compared with next generation sequencing (NGS) (Figure 6) (38-40). Specificity was 100% in detecting fusions of ROS1, RET, NTRK2/3, and MET exon 14 skipping, while 98% was reported for ALK (38-40). The assay is very versatile, successfully performing with biopsy and cell blocks even when the sample has 5% of tumor content or cytology smears with at least 300 cells and extracted RNA of 20 ng (39).
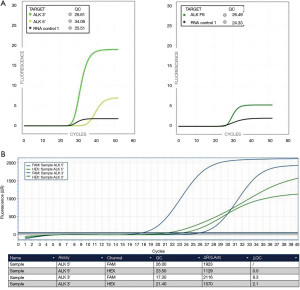
Next generation sequencing
In agreement with international recommendations, ALK testing is just one among several other druggable targets that should be tested in the current clinical practice, including mutations (EGFR, BRAF, MET, KRAS, HER2) and rearrangements (ROS1, RET, NTRK1/2/3) (41-43). Then, it is essential to have a throughout technology detecting all predictive molecular biomarkers from the same tumor sample. The development of fusion gene panels available into NGS platforms is becoming relatively cost-effective and this technology is becoming the gold standard permitting to cover all the predictive molecular biomarkers at once (44-46).
Although preanalytical factors (including the expertise of molecular biologist, the sample type, the timing and type of fixation) and postanalytical factors may significantly impact on the quality of the final results, NGS has been suggested as the new “gold-standard” in profiling NSCLC for predictive molecular determinations, including ALK gene fusions (46).
Several studies using amplicon-based or hybrid capture-based NGS demonstrated a very high sensitivity (100%) and specificity (>98%) when compared with IHC and/or FISH in ALK-positive NSCLC. Most importantly, NGS permits to discover novel and complex ALK gene fusion partners not detected by FISH and/or RT-PCR (Figure 7) (46).
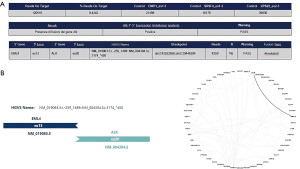
Interestingly, in a recent real-world ALK biomarker testing of patients with NSCLC in the United States, among 60,025 eligible patients, tumors from 36,691 (61.1%) patients were tested for ALK rearrangements, with 1,042 (2.8%) positive analyses (47). Of note, the rate of ALK testing increased from 33.1% in 2011 to 73.0% in 2019. ALK testing rates increased over time for patients either with non-squamous (41.6–81.6%) and squamous histology (13.6–50.4%) (47). The proportion of tests performed using FISH declined from 83.8% in 2012 to 32.1% in 2019. Conversely, the proportion of tests performed using NGS increased from 0.2% in 2011 to 52.2% in 2019. Again, the proportion of ALK tests performed using liquid biopsy increased from 0.1% in 2011 to 28.2% in 2019, whereas the use of tissue samples decreased from 98.0% in 2011 to 71.2% in 2019 (47). Indeed, the identification of actionable oncogenic drivers, including ALK gene fusions, on liquid biopsy could represent another method to investigate an alternative source of tumor tissue, particularly when patients present with a high tumor burden. Although ALK rearrangements on liquid biopsy does not represent a real-life practice and may lead to false negative results due to insufficient sensitivity, hybrid-capture NGS on plasma circulating free DNA has demonstrated the feasibility of this approach in determining EML4::ALK variants in clinical trials (48).
Since the discovery of EML4::ALK rearrangement, several gene fusions (e.g., ROS1, NTRK1-3, RET, NRG1, NUT, FGFR1, FGFR2, MET, BRAF, EGFR, SMARCA4) have been identified in lung cancer, also increasing the number of fusion partner genes. These findings, leading to the development of new and promising therapies, mandated the development and implementation of dedicated, comprehensive gene fusions detection methods (38,49). Sequential approaches have been developed using anchored, multiplexed, PCR-based targeted RNA sequencing (RNA NGS) when no oncogenic drivers alterations were detected by DNA-based targeted cancer hotspot NGS, and this strategy was proven particularly efficient in smoking-associated NSCLC (50).
Therapy
Defining ALK as a targetable oncogene: historical evidence
As mentioned in the section dedicated to the biology of ALK fusions in NSCLC, their first evidence dates back to 2007. It is also in the same year that we witnessed the first proofs of the possibility of targeted ALK inhibition. The apparently immediate availability of crizotinib (initially known as PF-2341066) was due to its initial development as a MET inhibitor (51). Crizotinib turned out to be active against ALK in models of ALCL (52), neuroblastoma and NSCLC (53). This initial preclinical evidence was rapidly followed by the pivotal phase I clinical trial PROFILE 1001. After defining the maximum tolerated dose of 250 mg twice daily in the dose escalating phase (54), dose expansion cohorts were dedicated to ALK-positive, ROS1-positive, and MET-amplified NSCLC (55-57). Positive evidence of the role of crizotinib in ALK-positive ALCL and other ALK-driven diseases was also produced (58-60). Besides providing the proof-of-concept of ALK inhibition in the field of precision medicine, crizotinib represented a crucial agent for the treatment of patients suffering from ALK-positive NSCLC, and still figures among the standards of care for ALCL, neuroblastoma, and inflammatory myofibroblastic tumor.
Crizotinib
Three phase 3 randomized trials defined the role of crizotinib in patients suffering from advanced, ALK-positive NSCLC either after platinum-based regimens [PROFILE 1007 (61)], or as the first-line therapy [PROFILE 1014 (62), PROFILE 1029 (63)]. These trials clearly reported the superiority of crizotinib over chemotherapy in terms of activity [objective response rate (ORR) and progression-free survival (PFS)], while overall survival (OS) did not differ significatively between the two arms. The final OS results of PROFILE 1014 confirmed that the apparent lack of efficacy benefit from crizotinib was due to the fact that the majority of patients initially randomized to chemotherapy received crizotinib at progression (64). The latter study confirmed that the longest survival benefit was obtained by patients receiving crizotinib followed by a new generation ALK TKI.
Resistance mechanisms to crizotinib and development of second-/third-generation inhibitors
The molecular analyses performed on patient samples progressing on crizotinib was frequently associated with functional studies to confirm the putative role of potential mechanism of resistance, and to suggest treatment strategies to restore sensitivity. The evidence of molecular mechanisms of resistance to crizotinib (and lately to new generation inhibitors) relied on case reports, case series and landscape studies, these latter providing a view on the resistance pattern most frequently observed across patients (65-71). Of note, the molecular analyses were mainly performed on tissue biopsies, as circulating tumor DNA (ctDNA) technologies were not fully developed at the time of the major studies of resistance to ALK inhibitors. Nevertheless, liquid biopsy has recently become a valid tool to assess resistance in this setting as well (72).
Schematically, the mechanism of resistance to crizotinib and ALK inhibitors can converge into four main types. Importantly, these categories can be seen applied to other targets and their corresponding inhibitors.
- On-target mechanisms of resistance: mutations occurring in the tyrosine kinase domain of ALK, or amplification of the rearranged copy of ALK (66,67). In line with other resistance mutations arising in the kinase domain of receptor tyrosine kinases, ALK mutations confer resistance by generating a steric hindrance, precluding the TKI from entering into its binding site, and/or by increasing the basal activity of the receptor itself, by increasing ATP-affinity. In contrast with EGFR, where the gatekeeper mutation T790M represent the almost unique mutation observed at resistance to first-/second-generation EGFR-TKI (73,74), ALK-TKI resistance mutations are more variegated. For the scope of this review we mention ALK G1202R as the most recalcitrant (i.e., conferring the highest levels of resistance in functional assays) and the most frequently observed at progression to second-generation ALK inhibitors (69,70).
- Off-target mechanisms of resistance: activation of signaling pathways by molecular events (mutations, fusions, amplifications, non-genetic functional hyper-activation) occurring as bypass (e.g., EGFR, MET activation) or downstream alterations (e.g., RAS, SRC mutations) (66,68,75-77).
- Mechanism globally related to cellular plasticity, likely implying epigenetic modifications acting through phenotypic changes such as epithelial-to-mesenchymal transition (69), acquisition of neuroendocrine phenotypes and small-cell lung cancer features (78,79), modulation of expression and the activity of the effectors and regulators of apoptotic processes (80-82).
- Of greatest importance in the field of ALK-positive NSCLC, central nervous system (CNS) progression. ALK-driven lung cancers have a peculiar brain tropism as shown by the high rate of CNS metastases at diagnosis, and CNS is a frequent site of progression to crizotinib, due to the limited capability of the first-generation TKI to cross the blood-brain barrier (83).
Second- (ceritinib, alectinib, brigatinib, ensartinib), and third- (lorlatinib) generation ALK inhibitors have been developed to satisfy particular characteristics, inspired by the resistance mechanisms just reported:
- Activity against mutations occurring in ALK tyrosine kinase domain. While second-generation inhibitors can overcome specific ALK mutations, lorlatinib is active against all the spectrum of resistance mutations, including the mentioned ALK G1202R (against which brigatinib as well could retain activity) (70,84). This statement is true for single ALK mutations, as “compound” mutations (i.e., multiple mutations occurring on the same ALK allele) confer resistance to lorlatinib (see below) (70,71).
- Higher potency against the wild-type ALK tyrosine kinase. Crizotinib likely exerts a sub-optimal inhibition of ALK fusions, thus in the case of a bypass such as EGFR, this latter can still confer resistance even in case of a mild activation, sustained by the ALK signaling partially maintained under crizotinib inhibition (66,68). A complete abrogation of ALK phosphorylation with new generation inhibitors limits the occurrence of bypass/downstream resistance to the occurrence of strong mechanisms, capable per se of maintaining signaling pathways.
- Increased ability to cross the blood-brain barrier, in order to achieve clinically meaningful concentration in the CNS.
- Higher ALK selectivity, sparing other kinases and limiting thus far off-target toxicities.
Across the three generations of ALK inhibitors, a progressive increase of the four characteristics have been achieved, with lorlatinib figuring as the most compelling agent due to its macrocyclic structure. Fourth-generation ALK inhibitors, designed to overcome lorlatinib resistance, have a more tolerable toxicity profile and have recently entered the initial phases of clinical development (see section “Moving beyond second- and third-generation ALK inhibitors”).
As mentioned above, the prolonged OS benefits observed in patients suffering from ALK-positive NSCLC were obtained with the sequential administration of crizotinib and new generation ALK TKI. This strategy, sustained by several clinical studies, has been challenged by the introduction of second- or third-generation ALK inhibitors given as upfront therapy (85). Considering that this latter strategy is supported by the main treatment guidelines (86-88), we report here the main results from the clinical trials evaluating alectinib, brigatinib or lorlatinib compared to crizotinib for the first-line treatment of advanced ALK-positive NSCLC. Of note, ceritinib has been evaluated as the upfront ALK inhibitor, providing a median PFS of 16.6 months (89), and is still included in current guidelines for the management of ALK-positive disease. Nevertheless, the sub-optimal comparator arm of ASCEND-4 study (platinum-based chemotherapy), the inferior PFS outcomes compared to the mentioned agents (Table 2) and the toxicity profile [then mitigated by the alternative doses-schedules proposed in ASCEND-8 (101)] limit the use of ceritinib in this setting. In addition, the second-generation inhibitor ensartinib has been proven superior to crizotinib in the phase 3 eXalt3 trial (102). Considering it has not been incorporated into clinical guidelines, we address readers to the corresponding publication in extenso of eXalt3 study for ensartinib data.
Table 2
Study characteristics and outcomes | Alectinib | Brigatinib | Lorlatinib | ||||
---|---|---|---|---|---|---|---|
ALEX (90,91) | J-ALEX (92-94) | ALESIA (95,96) | ALTA-1L (97,98) | CROWN (99,100) | |||
Enrollment | Global | Japan | Asia | Global | Global | ||
Patients | 303 | 207 | 187 | 275 | 296 | ||
Primary endpoint | Investigator-assessed PFS | IRF-assessed PFS | Investigator-assessed PFS | Blinded IRC-assessed PFS | Blinded IRC-assessed PFS | ||
Investigator-assessed median PFS (95% CI) | Alectinib 34.8 (17.7–NE) months | * | Alectinib 41.6 (33.1–58.9) months | Brigatinib 30.8 (21.3–40.6) months | Median FU: 37 months | ||
Crizotinib 10.9 (9.1–12.9) months | Crizotinib 11.1 (9.1–18.4) months | Crizotinib 9.2 (7.4–12.7) months | Lorlatinib NR (NR-NR) | ||||
Crizotinib 9.1 (7.4–10.9) months | |||||||
Investigator-assessed PFS (95% CI) | HR 0.43 (0.32–0.58); P<0.0001 | * | HR 0.33 (0.23–0.49) | HR 0.43 (0.31–0.58); P<0.0001 | HR 0.19 (0.13–0.27) | ||
IRC/IRF-assessed median PFS (95% CI) | * | Alectinib 34.1 (22.1–NE) months | * | Brigatinib 24.0 (18.5–43.2) months | Median FU: 37 months | ||
Crizotinib 10.2 (8.3–12.0) months | Crizotinib 11.1 (9.1–13.0) months | Lorlatinib NR (NR–NR) | |||||
Crizotinib 9.3 (7.6–11.1) | |||||||
IRC/IRF-assessed PFS (95% CI) | * | HR 0.37 (0.26–0.52) | * | HR 0.48 (0.35–0.66); P<0.0001 | HR 0.48 (0.35–0.66); P<0.0001 | ||
Median OS follow-up | 48 months | 68 months | 61 months | 40 months | NA (immature data) | ||
Median OS (95% CI) | Alectinib NR | Alectinib NE (70.6 months–NE) | Alectinib NE (NE–NE) | Brigatinib NR | NA (immature data) | ||
Crizotinib 57.4 (34.6–NR) months | Crizotinib NE (68.5 months–NE) | Crizotinib NE (45.5 months–NE) | Crizotinib NR | ||||
OS (95% CI) | HR 0.67 (0.46–0.98); P=0.0376 | HR 1.03 (0.67–1.58); P=0.9105 | HR 0.60 (0.37–0.99) | HR 0.81 (0.53–1.22); P=0.305 | NA (immature data) | ||
Landmark OS analyses (95% CI) | 3-year OS: | 5-year OS: | 5-year OS: | 3-year OS: | NA (immature data) | ||
Alectinib 67.0% (59.1–74.8%) | Alectinib 60.9% (51.4–70.3%) | Alectinib 66.4% (57.9–74.9%) | Brigatinib 71% (62–78%) | ||||
Crizotinib 57.0% (42.1–65.9%) | Crizotinib 64.1% (54.9–73.4%) | Crizotinib 56.0% (43.0–69.1%) | Crizotinib 68% (59–75%) | ||||
4-year OS: | 4-year OS: | ||||||
Alectinib 65.3% (55.3–73.3%) | Brigatinib 66% (56–74%) | ||||||
Crizotinib 51.2% (42.1–60.3%) | Crizotinib 60% (51–68%) | ||||||
5-year OS: | |||||||
Alectinib 62.5% (54.3–70.8%) | |||||||
Crizotinib 45.5% (33.6–57.4%) |
In all studies with the exception of J-ALEX, patients were stratified at randomization for the presence of baseline brain metastases. *, data published in the first reports of the corresponding trials, with short follow-up. All the studies had a 1:1 randomization with the exception of ALESIA (2:1). ALK, anaplastic lymphoma kinase; PFS, progression-free survival; IRF, independent review facility; IRC, independent review committee; HR, hazard ratio; 95% CI, 95% confidence interval; NE, not evaluable; FU, follow-up; NR, not reached; OS, overall survival; NA, not available.
Activity, efficacy and safety of next-generation inhibitors compared to crizotinib in head-to-head comparisons
Evidence and considerations upon ORR, PFS and OS
Alectinib, brigatinib and lorlatinib have been evaluated as the first ALK inhibitor (a minority of patients having received chemotherapy before) administered in patients suffering from ALK-positive NSCLC in five phase 3 studies, sharing crizotinib as the comparator arm (Table 2). Alectinib was tested in ALEX, J-ALEX and ALESIA, brigatinib in ALTA-1L, lorlatinib in CROWN (90,92,95,97,99). The ultimate goal of the strategy of having a new-generation ALK inhibitors upfront, compared to a sequential approach, is to provide longer survival outcomes with a good tolerability profile. Dealing with the first readout of activity, it is worth considering that ORR is still very high with crizotinib (up to 79% in J-ALEX). Still considering the variability between radiological assessments provided by investigators or the independent review committees, ORR are numerically higher with new generation inhibitors (up to 92% with alectinib in J-ALEX). Despite a potential impact on an increased depth of response obtained with new inhibitors, their intracranial activity is likely the major player in the higher ORR. The activity of ALK inhibitors in the ALEX trial is representative of these observations, as the investigator-assessed ORR in the groups with brain metastases is respectively 76.6% and 65.5% with alectinib (n=64) and crizotinib (n=58). In patients without baseline CNS involvement, the two inhibitors obtained overlapping activity: 79.5% in the alectinib arm (n=88) and 78.5% in the crizotinib one (n=93) (48). Besides the increased activity on brain lesions, it is difficult to envisage that novel inhibitors could rescue patients with primary resistance to crizotinib, occurring nevertheless in a minority of cases.
The crucial role of new generation inhibitors is represented by their prolonged PFS compared to crizotinib. Narrowing the range of resistance mechanisms, next-generation ALK TKI eventually turn out in a delayed occurrence of disease progression. Longer PFS (the endpoint of all the studies) was observed across all the trials, both in terms of statistical significance and of clinical relevance.
The benefit in OS, the final clinical readout of interest, is challenging to evaluate across these studies for two main reasons. First, the frequent exposure to next-generation inhibitors at progression to crizotinib for patients initially randomized in the control arms, and the fact that studies were not powered for OS, having been designed with PFS as endpoint. The OS data confirm how the prognosis of patients have evolved since the introduction of ALK inhibitor, as the only median OS reported thus far is for the crizotinib arm in ALEX trial, close to 5 years, whereas median OS is still unreached/not evaluable in the remaining treatment arms across trials (still considering the different follow-up periods) (91).
The best treatment strategy (sequential use of crizotinib and novel inhibitors versus novel inhibitors upfront) to be adopted in patients suffering from ALK-positive NSCLC has been a matter of discussion in the last years (85). Despite the methodological challenges in interpreting the OS data in ALEX, J-ALEX, ALESIA, ALTA-1L and CROWN trials (Table 2), the clinically meaningful increase in PFS, the activity against CNS disease and the better tolerability profiles favor the upfront use of the novel inhibitors.
Molecular features potentially impacting the activity of ALK inhibitors in this setting have been investigated. The type of EML4 variant (see section “ALK fusions in NSCLC: from structure to function” and Figure 1), as well as the co-occurrence of mutations in TP53 and CDKN2A/B tumor suppressor genes, have been shown to affect the prognosis of patients treated with ALK inhibitors in this setting (48,98,103,104). Nevertheless, these aspects do not harbor a predictive role, that could be of major clinical usefulness in suggesting which patient could benefit from a given treatment strategy/precise inhibitor on the basis of a wide molecular portrait.
Intracranial activity and efficacy
As anticipated above, the intracranial activity of new generation ALK inhibitors plays a crucial role in improving patient outcomes. There is a major difference in the intracranial response obtained with novel inhibitors or crizotinib in case of baseline brain involvement (Table 3). With the exception of ALEX trial and the pending results of CROWN, the presence of baseline brain metastases does not even represent a prognostic factor in patients treated with alectinib and brigatinib. All the publications of the trials contain clinically relevant information concerning parameters such as time to intracranial progression in patients with or without baseline CNS involvement, and inhibitors efficacy according to previous brain radiotherapy (93,96,98,100,105,107). As a summary, it can be stated that novel generation inhibitors prevent the onset of intracranial disease in patients without baseline CNS metastases. Further insights on these aspects, of clear clinical interest, go beyond the scope of this review, and readers are invited to refer to the specific publications.
Table 3
Study characteristics and outcomes | Alectinib | Brigatinib | Lorlatinib | |||||||||
---|---|---|---|---|---|---|---|---|---|---|---|---|
ALEX (90,91,105) | J-ALEX (92,94) | ALESIA (95,96) | ALTA-1L (98,106) | CROWN (100) | ||||||||
IRC/IRF-assessed intracranial ORR | Alectinib 59% (38/64) | NA | Alectinib 73% (32/44) | Brigatinib 66% (31/47) | Lorlatinib 65% (24/37) | |||||||
Crizotinib 26% (15/58) | Crizotinib 22% (5/23) | Crizotinib 14% (7/49) | Crizotinib 18% (7/39) | |||||||||
Baseline brain metastases in the new-generation ALK inhibitor arm | Yes (N=64) | No (N=88) | Yes (N=14) | No (N=89) | Yes (N=44) | No (N=81) | Yes (N=40) | No (N=97) | Yes (N=37) | No (N=112) | ||
Median PFS (95% CI) | 25.4 months (9.2⎯NE) | 38.6 months (22.4⎯NE) | NA | 42.3 months (27.8⎯60.7) | 41.6 months (29.5⎯64.9) | 24.0 months (18.4⎯NR) | 24.0 months (15.7⎯NR) | NR (18.2 months⎯NR) | NR (NR⎯NR) | |||
PFS crizotinib comparator arm (95% CI) | HR 0.37 (0.23⎯0.58) | HR 0.46 (0.31⎯0.68) | HR 0.08 (0.01–0.61) | HR 0.39 (0.23–0.64) | HR 0.17 (0.09⎯0.33) | HR 0.45 (0.29⎯0.71) | HR 0.25 (0.14⎯0.46); P<0.0001 | HR 0.65 (0.44⎯0.97); P=0.03 | HR 0.21 (0.10–0.44) | HR 0.29 (0.19–0.44) | ||
Median OS | NA | 53.9 | NE | NE | NE | NR | NR | NA (immature data) | ||||
OS crizotinib comparator arm (95% CI) | HR 0.58 (0.34⎯1.00); P=0.0477 | HR 0.76 (0.45⎯1.26); P=0.2851 | 1.56 (0.64-3.80) | 0.96 (0.58⎯1.57) | HR 0.40 (0.19⎯0.85) | HR 0.81 (0.42⎯1.55) | HR 0.43 (0.21–0.89); P=0.020 | HR 1.16 (0.69–1.93); P=0.603 | NA (immature data) | |||
Interaction test P=0.4677 | ||||||||||||
Landmark OS analyses | NA | 5-year OS 42.9% | 5-year OS 63.7% | 5-year OS 63.6% | 5-year OS 67.8% | 3-year OS 74% (95% CI: 57–85%) | 3-year OS 70% (95% CI: 59–78%) | NA (immature data) | ||||
4-year OS 71% (95% CI: 53–83%) | 4-year OS 64% (95% CI: 52–74%) |
The PFS data reported for studies refer either to investigator assessment (ALEX and ALESIA), IRF (independent review facility, J-ALEX) or BIRC (blinded independent review committee, ALTA-1L and CROWN). The discrepancy between patients evaluated for brigatinib intracranial ORR (n=47) and survival analyses (n=40) in ALTA-1L is explained considering that the 47 patients were considered positive for brain metastases at baseline by BIRC, and the 40 in the PFS/OS analyses correspond to the ones having baseline brain metastases according to investigator assessment. In all studies with the exception of J-ALEX, patients were stratified at randomization for the presence of baseline brain metastases. All the studies had a 1:1 randomization with the exception of ALESIA (2:1). ALK, anaplastic lymphoma kinase; IRC, independent review committee; IRF, independent review facility; ORR, objective response rate; PFS, progression-free survival; 95% CI, 95% confidence interval; HR, hazard ratio; NE, not evaluable; NA, not available; NR, not reached; OS, overall survival.
Safety considerations
Overall, new generation inhibitors are considered safer compared to crizotinib. Their global safety profiles are reported in Table 4. According to the mentioned clinical trials, grade 3–4 adverse events occur in 37% to 76% of the patients during treatment with new inhibitors; dose reductions (20–44%), interruption (26–72%) and treatment discontinuations (7–14%) are not anecdotical. Despite being frequently represented by laboratory abnormalities, treating physicians should be aware of the expected toxicities of each novel ALK inhibitor, in order to provide an early recognition and management. Grade 3–4 increase in blood levels of creatine phospho-kinase occur in up to 26% of the cases in patients receiving brigatinib, that can cause increase in blood lipase and amylase as well. Of note, no case of pancreatitis and no grade ≥3 myalgia or musculoskeletal pain were recorded (98). Lorlatinib is known to increase the blood levels of lipids and weight gain, again without a clear correlation with an increased risk of cardiovascular adverse events. The CNS toxic effects of lorlatinib, mainly in the areas of cognitive and mood effects, were rarely reported as grade 3–4 (107). Nevertheless, their frequent occurrence (39% across all grades) points out the relevance of mild but symptomatic toxicities, even more in patients who hopefully receive targeted therapies for years. Recommendations for the daily management of adverse events from novel ALK inhibitors have been recently published (108-111).
Table 4
Toxicities | Alectinib | Brigatinib | Lorlatinib | ||||
---|---|---|---|---|---|---|---|
ALEX (91) | J-ALEX (94) | ALESIA (96) | ALTA-1L (98) | CROWN (100) | |||
Patients | 152 | 103 | 125 | 136 | 149 | ||
Serious AE | 38.8% | 27.2% | 28% | NA | 38% | ||
Grade 3–5 AE | 52% | 36.9% | 48% | 70% (G3–4) | 76% (G3–4) | ||
Fatal AE | 4.6% | 0 | 4% | 8% | 7% | ||
Treatment-related, fatal AE | NA | 0 | NA | 0 | 1% | ||
AE leading to treatment discontinuation | 14.5% | 11.7% | 11.2% | 13% | 7% | ||
AE leading to dose reduction | 20.4% | NA | 26.4% | 44% | 21% | ||
AE leading to dose interruption | 26.3% | 34% | 26.4% | 72% | 56% | ||
Top G3–4 AEs | Anemia 5.9% | Blood CPK increased 4.9% | Weight increased 8.8% | Blood CPK increased 26% | Hypertriglyceridaemia 23% | ||
AST increased 5.3% | Interstitial lung disease 4.9% | Blood CPK increased 6.4% | Lipase increased 15% | Hypercholesterolaemia 19% | |||
ALT increased 4.6% | Rash maculopapular 2.9% | ALT increased 2.4% | Hypertension 14% | Weight gain 20% | |||
Urinary tract infection 3.9% | Neutrophil count decreased 1.9% | Nausea 0.8% | Amylase increased 6% | Hypertension 11% | |||
Blood CPK increase 3.3% | QT prolonged 1.9% | Pneumonia 5% | Cardiovascular adverse events 7% (including blood CPK increase) | ||||
CNS adverse events 5% |
ALK, anaplastic lymphoma kinase; AE, adverse event; AST, aspartate transaminase; ALT, alanine transaminase; CPK, creatine phospho-kinase; CNS, central nervous system.
Current clinical scenario of ALK inhibition in NSCLC
The improvement in the clinical management of ALK-positive NSCLC with sequential administration of different generations of ALK inhibitors observed in the “crizotinib era” vouches for the application of the strategy with novel agents. The concepts of treatment “beyond progression” in case of mild, asymptomatic radiological disease progression and of local therapy in case of isolated progression are still valid (112), as the goal is to maintain ALK inhibition as long as possible.
Dealing with the sequential administration of novel ALK-TKI, brigatinib has been specifically evaluated in patients progressing after ceritinib and alectinib (113,114), but translating the results in the current treatment scenario is challenging, as the patient populations included patients initially treated with crizotinib. For the same reason, evaluating lorlatinib outcomes in the precise patient population pre-treated with alectinib or brigatinib only is not straightforward, due to the relative heterogeneity of patients included in the phase 2 study studying lorlatinib in this setting (115).
It can be estimated nevertheless that alectinib-pretreated patients obtained an ORR and median PFS of approximately 40% and 5–6 months, respectively, with more optimistic results from a recent retrospective study (116). The eminent activity of the third-generation agent in the case of CNS disease (including meningeal metastases) sustain its utilization at progression to second-generation inhibitors (117,118). Even if not supported by formal proofs, the concomitant association of chemotherapy and new generation ALK inhibitors has been reported in some experience, likely with the goal of maintaining an increased control or prevention of CNS disease (119,120).
Moving beyond second- and third-generation ALK inhibitors
Whether in the setting of a sequential treatment encompassing crizotinib, or after an upfront treatment with a second-generation ALK inhibitor, or in the case of the adoption of CROWN results in the clinical setting, lorlatinib represents nowadays the last bulwark of targeted agents in patients with ALK-positive NSCLC.
From a clinical standpoint, after lorlatinib failure, treatment options turn to chemotherapy. Similarly to the EGFR-mutated NSCLC field, single-agent anti-PD-1/PD-L1 agents have been proven as globally inactive in ALK-positive NSCLC (121), excluded therefore from the large majority of trials evaluating chemo-immunotherapy regimens. Nevertheless, IMpower150 allowed the inclusion of oncogene-driven diseases. In its EGFR-mutant population, the potential synergy of the double blockade of PD-1/PD-L1 and angiogenesis with atezolizumab and bevacizumab, respectively, combined with carboplatin and paclitaxel chemotherapy, is still under debate (122). ALK-positive disease tends to be approached in line with the EGFR-driven one and the evoked combination therapy is mentioned in current guidelines (87). Nevertheless, it should be noted that in IMpower150 only 13 patients with ALK-rearranged lung cancer were treated with the four-drug regimen (123). Still with the limitation of a low number of patients, in the GFPC 06-2018 trial, nine patients received the four drugs, obtaining median PFS and OS of 7.3 and 16.8 months, respectively (124). Treatment decision in the post-lorlatinib setting (chemotherapy +/− atezolizumab and bevacizumab) should therefore take into account the limited strength of the supporting evidence, and the tolerability of a four-drug regimen in a setting of pre-treated patients.
The mentioned substantial improvement in the survival outcomes of patients with ALK-positive NSCLC through the last decade serve as an incitement to find additional targeted agents beyond lorlatinib. Fourth-generation agents such as TPX-0131 and NVL-655 have been developed to maintain the characteristics of an optimal ALK inhibitor, and to be active against compound mutations (i.e., double mutations responsible for resistance to lorlatinib, when administered after previous targeted agents) (125). Early clinical studies are ongoing and initial data are eagerly awaited.
Treatment considerations for locally advanced and early-stage ALK-positive NSCLC
The activity and efficacy of ALK inhibitors in the advanced-metastatic disease setting support their putative role in locally-advanced and early disease stages, in line with what has been recently shown in EGFR-driven NSCLC (126,127). Experiences of neo-adjuvant ALK inhibitors have been reported, and this approach is currently been evaluated in clinical trials, where pathological response serves as a surrogate marker of clinical activity (128). Compared to the advanced disease setting, assessing the benefit of an adjuvant approach could be more challenging, having disease-free survival and OS as ultimate goals. Nevertheless, the marked activity of new generation of ALK inhibitors would likely guarantee their competence in preventing/delaying disease onset after surgical treatment.
Of note, just before the final acceptance of this manuscript, the study ALINA was presented at European Society for Medical Oncology (ESMO) 2023 congress. ALINA is a phase 3 study, randomizing patients with resected, ALK-positive, stage IB-IIIA lung cancer (per UICC/AJCC 7th edition), to receive adjuvant chemotherapy or alectinib for two years. The trial met its primary endpoint of disease-free survival, with 94% and 88% of the patients in the alectinib arm not experiencing tumor recurrence at two at three years from randomization, respectively (129).
The limited activity of immunotherapy in advanced disease, and the preliminary proofs of the limited impact of durvalumab in patients with EGFR-mutant disease undergoing chemo-radiotherapy (130,131) suggest this could be the case for ALK-rearranged cases. Moreover, the risk of toxicities exacerbated by the sequential use of anti-PD-1/PD-L1 agents and ALK inhibitors should be taken into account when balancing the risk/befit ratio in treatment decision making (121).
These reflections on the potential incorporation of ALK inhibitors in the early/locally-advanced disease stages, and the potential exclusion of immunotherapy approaches, would require ALK testing by IHC in these settings, a procedure that could be easily implemented in the workflow of pathology diagnostic (see section “Molecular diagnostics for the detection of ALK fusions in NSCLC”).
Conclusions
This review aimed to retrace the history of ALK fusions in NSCLC, from their discovery to the latest therapeutic developments. The improvements in the clinical outcomes obtained in the last two decades for patients suffering from ALK-positive lung tumors are the results of the fruitful enlacement between the understanding of biological processes leading to resistance to available ALK inhibitors, and clinical research efforts. We hope that this bond, serving as a model across oncogene-driven tumors, will be maintained in the future, to guarantee the constant improvement of patient outcomes.
Acknowledgments
Funding: None.
Footnote
Provenance and Peer Review: This article was commissioned by the Guest Editors (Fabrizio Tabbò, Umberto Malapelle, Maria Lucia Reale and Angela Listì) for the series “How to Detect and Treat NSCLC Patients with Oncogenic Fusions” published in Precision Cancer Medicine. The article has undergone external peer review.
Peer Review File: Available at https://pcm.amegroups.com/article/view/10.21037/pcm-23-18/prf
Conflicts of Interest: All authors have completed the ICMJE uniform disclosure form (available at https://pcm.amegroups.com/article/view/10.21037/pcm-23-18/coif). The series “How to Detect and Treat NSCLC Patients with Oncogenic Fusions” was commissioned by the editorial office without any funding or sponsorship. M.T. received speakers’ and consultants’ fee from Pfizer, Novartis, Roche, Otsuka and Takeda. L.F. declares an institutional research contract with Nuvalent. The authors have no other conflicts of interest to declare.
Ethical Statement:
Open Access Statement: This is an Open Access article distributed in accordance with the Creative Commons Attribution-NonCommercial-NoDerivs 4.0 International License (CC BY-NC-ND 4.0), which permits the non-commercial replication and distribution of the article with the strict proviso that no changes or edits are made and the original work is properly cited (including links to both the formal publication through the relevant DOI and the license). See: https://creativecommons.org/licenses/by-nc-nd/4.0/.
References
- De Santis S, Monaldi C, Mancini M, et al. Overcoming Resistance to Kinase Inhibitors: The Paradigm of Chronic Myeloid Leukemia. Onco Targets Ther 2022;15:103-16. [Crossref] [PubMed]
- Thai AA, Solomon BJ, Sequist LV, et al. Lung cancer. Lancet 2021;398:535-54. [Crossref] [PubMed]
- Paez JG, Jänne PA, Lee JC, et al. EGFR mutations in lung cancer: correlation with clinical response to gefitinib therapy. Science 2004;304:1497-500. [Crossref] [PubMed]
- Lynch TJ, Bell DW, Sordella R, et al. Activating mutations in the epidermal growth factor receptor underlying responsiveness of non-small-cell lung cancer to gefitinib. N Engl J Med 2004;350:2129-39. [Crossref] [PubMed]
- Soda M, Choi YL, Enomoto M, et al. Identification of the transforming EML4-ALK fusion gene in non-small-cell lung cancer. Nature 2007;448:561-6. [Crossref] [PubMed]
- Zhao S, Li J, Xia Q, et al. New perspectives for targeting therapy in ALK-positive human cancers. Oncogene 2023;42:1959-69. [Crossref] [PubMed]
- Takeyasu Y, Okuma HS, Kojima Y, et al. Impact of ALK Inhibitors in Patients With ALK-Rearranged Nonlung Solid Tumors. JCO Precis Oncol 2021;5:PO.20.00383.
- Morris SW, Kirstein MN, Valentine MB, et al. Fusion of a kinase gene, ALK, to a nucleolar protein gene, NPM, in non-Hodgkin's lymphoma. Science 1994;263:1281-4. Correction appears in Science 1995;267:316-7.
- Rikova K, Guo A, Zeng Q, et al. Global survey of phosphotyrosine signaling identifies oncogenic kinases in lung cancer. Cell 2007;131:1190-203. [Crossref] [PubMed]
- Xiang Y, Zhang S, Fang X, et al. Therapeutic Advances of Rare ALK Fusions in Non-Small Cell Lung Cancer. Curr Oncol 2022;29:7816-31. [Crossref] [PubMed]
- Pulford K, Morris SW, Turturro F. Anaplastic lymphoma kinase proteins in growth control and cancer. J Cell Physiol 2004;199:330-58. [Crossref] [PubMed]
- Pulford K, Lamant L, Espinos E, et al. The emerging normal and disease-related roles of anaplastic lymphoma kinase. Cell Mol Life Sci 2004;61:2939-53. [Crossref] [PubMed]
- Palmer RH, Vernersson E, Grabbe C, et al. Anaplastic lymphoma kinase: signalling in development and disease. Biochem J 2009;420:345-61. [Crossref] [PubMed]
- Thunnissen E, Bubendorf L, Dietel M, et al. EML4-ALK testing in non-small cell carcinomas of the lung: a review with recommendations. Virchows Arch 2012;461:245-57. [Crossref] [PubMed]
- Camidge DR, Kono SA, Flacco A, et al. Optimizing the detection of lung cancer patients harboring anaplastic lymphoma kinase (ALK) gene rearrangements potentially suitable for ALK inhibitor treatment. Clin Cancer Res 2010;16:5581-90. [Crossref] [PubMed]
- Lindeman NI, Cagle PT, Aisner DL, et al. Updated Molecular Testing Guideline for the Selection of Lung Cancer Patients for Treatment With Targeted Tyrosine Kinase Inhibitors: Guideline From the College of American Pathologists, the International Association for the Study of Lung Cancer, and the Association for Molecular Pathology. J Mol Diagn 2018;20:129-59. [Crossref] [PubMed]
- Rodig SJ, Mino-Kenudson M, Dacic S, et al. Unique clinicopathologic features characterize ALK-rearranged lung adenocarcinoma in the western population. Clin Cancer Res 2009;15:5216-23. [Crossref] [PubMed]
- Shaw AT, Yeap BY, Mino-Kenudson M, et al. Clinical features and outcome of patients with non-small-cell lung cancer who harbor EML4-ALK. J Clin Oncol 2009;27:4247-53. [Crossref] [PubMed]
- Inamura K, Takeuchi K, Togashi Y, et al. EML4-ALK lung cancers are characterized by rare other mutations, a TTF-1 cell lineage, an acinar histology, and young onset. Mod Pathol 2009;22:508-15. [Crossref] [PubMed]
- Wong DW, Leung EL, So KK, et al. The EML4-ALK fusion gene is involved in various histologic types of lung cancers from nonsmokers with wild-type EGFR and KRAS. Cancer 2009;115:1723-33. [Crossref] [PubMed]
- Gainor JF, Varghese AM, Ou SH, et al. ALK rearrangements are mutually exclusive with mutations in EGFR or KRAS: an analysis of 1,683 patients with non-small cell lung cancer. Clin Cancer Res 2013;19:4273-81. [Crossref] [PubMed]
- Baldi L, Mengoli MC, Bisagni A, et al. Concomitant EGFR mutation and ALK rearrangement in lung adenocarcinoma is more frequent than expected: report of a case and review of the literature with demonstration of genes alteration into the same tumor cells. Lung Cancer 2014;86:291-5. [Crossref] [PubMed]
- Cai W, Lin D, Wu C, et al. Intratumoral Heterogeneity of ALK-Rearranged and ALK/EGFR Coaltered Lung Adenocarcinoma. J Clin Oncol 2015;33:3701-9. [Crossref] [PubMed]
- Won JK, Keam B, Koh J, et al. Concomitant ALK translocation and EGFR mutation in lung cancer: a comparison of direct sequencing and sensitive assays and the impact on responsiveness to tyrosine kinase inhibitor. Ann Oncol 2015;26:348-54. [Crossref] [PubMed]
- Marchetti A, Barberis M, Papotti M, et al. ALK rearrangement testing by FISH analysis in non-small-cell lung cancer patients: results of the first italian external quality assurance scheme. J Thorac Oncol 2014;9:1470-6. [Crossref] [PubMed]
- Marchetti A, Ardizzoni A, Papotti M, et al. Recommendations for the analysis of ALK gene rearrangements in non-small-cell lung cancer: a consensus of the Italian Association of Medical Oncology and the Italian Society of Pathology and Cytopathology. J Thorac Oncol 2013;8:352-8. [Crossref] [PubMed]
- Wynes MW, Sholl LM, Dietel M, et al. An international interpretation study using the ALK IHC antibody D5F3 and a sensitive detection kit demonstrates high concordance between ALK IHC and ALK FISH and between evaluators. J Thorac Oncol 2014;9:631-8. [Crossref] [PubMed]
- Conde E, Suárez-Gauthier A, Benito A, et al. Accurate identification of ALK positive lung carcinoma patients: novel FDA-cleared automated fluorescence in situ hybridization scanning system and ultrasensitive immunohistochemistry. PLoS One 2014;9:e107200. [Crossref] [PubMed]
- Yi ES, Boland JM, Maleszewski JJ, et al. Correlation of IHC and FISH for ALK gene rearrangement in non-small cell lung carcinoma: IHC score algorithm for FISH. J Thorac Oncol 2011;6:459-65. [Crossref] [PubMed]
- Sholl LM, Weremowicz S, Gray SW, et al. Combined use of ALK immunohistochemistry and FISH for optimal detection of ALK-rearranged lung adenocarcinomas. J Thorac Oncol 2013;8:322-8. [Crossref] [PubMed]
- Teixidó C, Karachaliou N, Peg V, et al. Concordance of IHC, FISH and RT-PCR for EML4-ALK rearrangements. Transl Lung Cancer Res 2014;3:70-4. [PubMed]
- Sun JM, Choi YL, Won JK, et al. A dramatic response to crizotinib in a non-small-cell lung cancer patient with IHC-positive and FISH-negative ALK. J Thorac Oncol 2012;7:e36-8. [Crossref] [PubMed]
- van der Wekken AJ, Pelgrim R, 't Hart N, et al. Dichotomous ALK-IHC Is a Better Predictor for ALK Inhibition Outcome than Traditional ALK-FISH in Advanced Non-Small Cell Lung Cancer. Clin Cancer Res 2017;23:4251-8. [Crossref] [PubMed]
- Canterbury CR, Fernandes H, Crapanzano JP, et al. ALK Gene Rearrangements in Lung Adenocarcinomas: Concordance of Immunohistochemistry, Fluorescence In Situ Hybridization, RNA In Situ Hybridization, and RNA Next-Generation Sequencing Testing. JTO Clin Res Rep 2021;2:100223. [Crossref] [PubMed]
- Zhang X, Zhou JG, Wu HL, et al. Diagnostic accuracy of PCR for detecting ALK gene rearrangement in NSCLC patients: A systematic review and meta-analysis. Oncotarget 2017;8:75400-10. [Crossref] [PubMed]
- Reguart N, Teixidó C, Giménez-Capitán A, et al. Identification of ALK, ROS1, and RET Fusions by a Multiplexed mRNA-Based Assay in Formalin-Fixed, Paraffin-Embedded Samples from Advanced Non-Small-Cell Lung Cancer Patients. Clin Chem 2017;63:751-60. [Crossref] [PubMed]
- Lira ME, Choi YL, Lim SM, et al. A single-tube multiplexed assay for detecting ALK, ROS1, and RET fusions in lung cancer. J Mol Diagn 2014;16:229-43. [Crossref] [PubMed]
- Hofman V, Heeke S, Bontoux C, et al. Ultrafast Gene Fusion Assessment for Nonsquamous NSCLC. JTO Clin Res Rep 2023;4:100457. [Crossref] [PubMed]
- Buglioni A, Caffes PL, Hessler MG, et al. Clinical Utility Validation of an Automated Ultrarapid Gene Fusion Assay for NSCLC. JTO Clin Res Rep 2022;3:100434. [Crossref] [PubMed]
- Leone A, Muscarella LA, Graziano P, et al. Robust Performance of the Novel Research-Use-Only Idylla GeneFusion Assay Using a Diverse Set of Pathological Samples with a Proposed 1-Day Workflow for Advanced NSCLC Evaluation. Cancers (Basel) 2022;15:292. [Crossref] [PubMed]
- Ettinger DS, Wood DE, Aisner DL, et al. Non-Small Cell Lung Cancer, Version 3.2022, NCCN Clinical Practice Guidelines in Oncology. J Natl Compr Canc Netw 2022;20:497-530. [Crossref] [PubMed]
- Isla D, Lozano MD, Paz-Ares L, et al. New update to the guidelines on testing predictive biomarkers in non-small-cell lung cancer: a National Consensus of the Spanish Society of Pathology and the Spanish Society of Medical Oncology. Clin Transl Oncol 2023;25:1252-67. [Crossref] [PubMed]
- Mitsudomi T, Tan D, Yang JC, et al. Expert Consensus Recommendations on Biomarker Testing in Metastatic and Nonmetastatic NSCLC in Asia. J Thorac Oncol 2023;18:436-46. [Crossref] [PubMed]
- Peled N, Palmer G, Hirsch FR, et al. Next-generation sequencing identifies and immunohistochemistry confirms a novel crizotinib-sensitive ALK rearrangement in a patient with metastatic non-small-cell lung cancer. J Thorac Oncol 2012;7:e14-6. [Crossref] [PubMed]
- Pekar-Zlotin M, Hirsch FR, Soussan-Gutman L, et al. Fluorescence in situ hybridization, immunohistochemistry, and next-generation sequencing for detection of EML4-ALK rearrangement in lung cancer. Oncologist 2015;20:316-22. [Crossref] [PubMed]
- Mosele F, Remon J, Mateo J, et al. Recommendations for the use of next-generation sequencing (NGS) for patients with metastatic cancers: a report from the ESMO Precision Medicine Working Group. Ann Oncol 2020;31:1491-505. [Crossref] [PubMed]
- Lin HM, Wu Y, Yin Y, et al. Real-world ALK Testing Trends in Patients With Advanced Non-Small-Cell Lung Cancer in the United States. Clin Lung Cancer 2023;24:e39-49. [Crossref] [PubMed]
- Camidge DR, Dziadziuszko R, Peters S, et al. Updated Efficacy and Safety Data and Impact of the EML4-ALK Fusion Variant on the Efficacy of Alectinib in Untreated ALK-Positive Advanced Non-Small Cell Lung Cancer in the Global Phase III ALEX Study. J Thorac Oncol 2019;14:1233-43. [Crossref] [PubMed]
- De Luca C, Pepe F, Iaccarino A, et al. RNA-Based Assay for Next-Generation Sequencing of Clinically Relevant Gene Fusions in Non-Small Cell Lung Cancer. Cancers (Basel) 2021;13:139. [Crossref] [PubMed]
- Cohen D, Hondelink LM, Solleveld-Westerink N, et al. Optimizing Mutation and Fusion Detection in NSCLC by Sequential DNA and RNA Sequencing. J Thorac Oncol 2020;15:1000-14. [Crossref] [PubMed]
- Zou HY, Li Q, Lee JH, et al. An orally available small-molecule inhibitor of c-Met, PF-2341066, exhibits cytoreductive antitumor efficacy through antiproliferative and antiangiogenic mechanisms. Cancer Res 2007;67:4408-17. [Crossref] [PubMed]
- Christensen JG, Zou HY, Arango ME, et al. Cytoreductive antitumor activity of PF-2341066, a novel inhibitor of anaplastic lymphoma kinase and c-Met, in experimental models of anaplastic large-cell lymphoma. Mol Cancer Ther 2007;6:3314-22. [Crossref] [PubMed]
- McDermott U, Iafrate AJ, Gray NS, et al. Genomic alterations of anaplastic lymphoma kinase may sensitize tumors to anaplastic lymphoma kinase inhibitors. Cancer Res 2008;68:3389-95. [Crossref] [PubMed]
- Clark JW, Camidge DR, Kwak EL, et al. Dose-escalation trial of the ALK, MET & ROS1 inhibitor, crizotinib, in patients with advanced cancer. Future Oncol 2020;16:4289-301. [Crossref] [PubMed]
- Kwak EL, Bang YJ, Camidge DR, et al. Anaplastic lymphoma kinase inhibition in non-small-cell lung cancer. N Engl J Med 2010;363:1693-703. [Crossref] [PubMed]
- Shaw AT, Ou SH, Bang YJ, et al. Crizotinib in ROS1-rearranged non-small-cell lung cancer. N Engl J Med 2014;371:1963-71. [Crossref] [PubMed]
- Camidge DR, Otterson GA, Clark JW, et al. Crizotinib in Patients With MET-Amplified NSCLC. J Thorac Oncol 2021;16:1017-29. [Crossref] [PubMed]
- Gambacorti-Passerini C, Messa C, Pogliani EM. Crizotinib in anaplastic large-cell lymphoma. N Engl J Med 2011;364:775-6. [Crossref] [PubMed]
- Mossé YP, Lim MS, Voss SD, et al. Safety and activity of crizotinib for paediatric patients with refractory solid tumours or anaplastic large-cell lymphoma: a Children's Oncology Group phase 1 consortium study. Lancet Oncol 2013;14:472-80. [Crossref] [PubMed]
- Gambacorti-Passerini C, Orlov S, Zhang L, et al. Long-term effects of crizotinib in ALK-positive tumors (excluding NSCLC): A phase 1b open-label study. Am J Hematol 2018;93:607-14. [Crossref] [PubMed]
- Shaw AT, Kim DW, Nakagawa K, et al. Crizotinib versus chemotherapy in advanced ALK-positive lung cancer. N Engl J Med 2013;368:2385-94. [Crossref] [PubMed]
- Solomon BJ, Mok T, Kim DW, et al. First-line crizotinib versus chemotherapy in ALK-positive lung cancer. N Engl J Med 2014;371:2167-77. [Crossref] [PubMed]
- Wu YL, Lu S, Lu Y, et al. Results of PROFILE 1029, a Phase III Comparison of First-Line Crizotinib versus Chemotherapy in East Asian Patients with ALK-Positive Advanced Non-Small Cell Lung Cancer. J Thorac Oncol 2018;13:1539-48. [Crossref] [PubMed]
- Solomon BJ, Kim DW, Wu YL, et al. Final Overall Survival Analysis From a Study Comparing First-Line Crizotinib Versus Chemotherapy in ALK-Mutation-Positive Non-Small-Cell Lung Cancer. J Clin Oncol 2018;36:2251-8. [Crossref] [PubMed]
- Choi YL, Soda M, Yamashita Y, et al. EML4-ALK mutations in lung cancer that confer resistance to ALK inhibitors. N Engl J Med 2010;363:1734-9. [Crossref] [PubMed]
- Katayama R, Shaw AT, Khan TM, et al. Mechanisms of acquired crizotinib resistance in ALK-rearranged lung Cancers. Sci Transl Med 2012;4:120ra17. [Crossref] [PubMed]
- Doebele RC, Pilling AB, Aisner DL, et al. Mechanisms of resistance to crizotinib in patients with ALK gene rearranged non-small cell lung cancer. Clin Cancer Res 2012;18:1472-82. [Crossref] [PubMed]
- Friboulet L, Li N, Katayama R, et al. The ALK inhibitor ceritinib overcomes crizotinib resistance in non-small cell lung cancer. Cancer Discov 2014;4:662-73. [Crossref] [PubMed]
- Gainor JF, Dardaei L, Yoda S, et al. Molecular Mechanisms of Resistance to First- and Second-Generation ALK Inhibitors in ALK-Rearranged Lung Cancer. Cancer Discov 2016;6:1118-33. [Crossref] [PubMed]
- Yoda S, Lin JJ, Lawrence MS, et al. Sequential ALK Inhibitors Can Select for Lorlatinib-Resistant Compound ALK Mutations in ALK-Positive Lung Cancer. Cancer Discov 2018;8:714-29. [Crossref] [PubMed]
- Recondo G, Mezquita L, Facchinetti F, et al. Diverse Resistance Mechanisms to the Third-Generation ALK Inhibitor Lorlatinib in ALK-Rearranged Lung Cancer. Clin Cancer Res 2020;26:242-55. [Crossref] [PubMed]
- Dagogo-Jack I, Rooney M, Lin JJ, et al. Treatment with Next-Generation ALK Inhibitors Fuels Plasma ALK Mutation Diversity. Clin Cancer Res 2019;25:6662-70. [Crossref] [PubMed]
- Sequist LV, Waltman BA, Dias-Santagata D, et al. Genotypic and histological evolution of lung cancers acquiring resistance to EGFR inhibitors. Sci Transl Med 2011;3:75ra26. [Crossref] [PubMed]
- Yu HA, Arcila ME, Rekhtman N, et al. Analysis of tumor specimens at the time of acquired resistance to EGFR-TKI therapy in 155 patients with EGFR-mutant lung cancers. Clin Cancer Res 2013;19:2240-7. [Crossref] [PubMed]
- Crystal AS, Shaw AT, Sequist LV, et al. Patient-derived models of acquired resistance can identify effective drug combinations for cancer. Science 2014;346:1480-6. [Crossref] [PubMed]
- Katayama Y, Yamada T, Tanimura K, et al. Adaptive resistance to lorlatinib via EGFR signaling in ALK-rearranged lung cancer. NPJ Precis Oncol 2023;7:12. [Crossref] [PubMed]
- Dagogo-Jack I, Yoda S, Lennerz JK, et al. MET Alterations Are a Recurring and Actionable Resistance Mechanism in ALK-Positive Lung Cancer. Clin Cancer Res 2020;26:2535-45. [Crossref] [PubMed]
- Caumont C, Veillon R, Gros A, et al. Neuroendocrine phenotype as an acquired resistance mechanism in ALK-rearranged lung adenocarcinoma. Lung Cancer 2016;92:15-8. [Crossref] [PubMed]
- Li H, Song T, Xu X. ALK-Positive Adenocarcinoma After Acquired Resistance to Lorlatinib and Transformation to SCLC: A Case Report. JTO Clin Res Rep 2023;4:100464. [Crossref] [PubMed]
- Tanimura K, Yamada T, Horinaka M, et al. Inhibition of c-Jun N-terminal kinase signaling increased apoptosis and prevented the emergence of ALK-TKI-tolerant cells in ALK-rearranged non-small cell lung cancer. Cancer Lett 2021;522:119-28. [Crossref] [PubMed]
- Tsuji T, Ozasa H, Aoki W, et al. YAP1 mediates survival of ALK-rearranged lung cancer cells treated with alectinib via pro-apoptotic protein regulation. Nat Commun 2020;11:74. [Crossref] [PubMed]
- Yanagimura N, Takeuchi S, Fukuda K, et al. STAT3 inhibition suppresses adaptive survival of ALK-rearranged lung cancer cells through transcriptional modulation of apoptosis. NPJ Precis Oncol 2022;6:11. [Crossref] [PubMed]
- Solomon BJ, Cappuzzo F, Felip E, et al. Intracranial Efficacy of Crizotinib Versus Chemotherapy in Patients With Advanced ALK-Positive Non-Small-Cell Lung Cancer: Results From PROFILE 1014. J Clin Oncol 2016;34:2858-65. [Crossref] [PubMed]
- Zou HY, Friboulet L, Kodack DP, et al. PF-06463922, an ALK/ROS1 Inhibitor, Overcomes Resistance to First and Second Generation ALK Inhibitors in Preclinical Models. Cancer Cell 2015;28:70-81. [Crossref] [PubMed]
- Recondo G, Facchinetti F, Olaussen KA, et al. Making the first move in EGFR-driven or ALK-driven NSCLC: first-generation or next-generation TKI?. Nat Rev Clin Oncol 2018;15:694-708. [Crossref] [PubMed]
- Owen DH, Singh N, Ismaila N, et al. Therapy for Stage IV Non-Small-Cell Lung Cancer With Driver Alterations: ASCO Living Guideline, Version 2022.2. J Clin Oncol 2023;41:e10-e20. [Crossref] [PubMed]
- Hendriks LE, Kerr KM, Menis J, et al. Non-oncogene-addicted metastatic non-small-cell lung cancer: ESMO Clinical Practice Guideline for diagnosis, treatment and follow-up. Ann Oncol 2023;34:358-76. [Crossref] [PubMed]
- NCCN Guidelines Non-Small Cell Lung Cancer (nccn.org) accessed the 09th March 2023. Available online: https://www.nccn.org/guidelines/guidelines-detail?category=1&id=1450
- Soria JC, Tan DSW, Chiari R, et al. First-line ceritinib versus platinum-based chemotherapy in advanced ALK-rearranged non-small-cell lung cancer (ASCEND-4): a randomised, open-label, phase 3 study. Lancet 2017;389:917-29. [Crossref] [PubMed]
- Peters S, Camidge DR, Shaw AT, et al. Alectinib versus Crizotinib in Untreated ALK-Positive Non-Small-Cell Lung Cancer. N Engl J Med 2017;377:829-38. [Crossref] [PubMed]
- Mok T, Camidge DR, Gadgeel SM, et al. Updated overall survival and final progression-free survival data for patients with treatment-naive advanced ALK-positive non-small-cell lung cancer in the ALEX study. Ann Oncol 2020;31:1056-64. [Crossref] [PubMed]
- Hida T, Nokihara H, Kondo M, et al. Alectinib versus crizotinib in patients with ALK-positive non-small-cell lung cancer (J-ALEX): an open-label, randomised phase 3 trial. Lancet 2017;390:29-39. [Crossref] [PubMed]
- Nakagawa K, Hida T, Nokihara H, et al. Final progression-free survival results from the J-ALEX study of alectinib versus crizotinib in ALK-positive non-small-cell lung cancer. Lung Cancer 2020;139:195-9. [Crossref] [PubMed]
- Hotta K, Hida T, Nokihara H, et al. Final overall survival analysis from the phase III J-ALEX study of alectinib versus crizotinib in ALK inhibitor-naïve Japanese patients with ALK-positive non-small-cell lung cancer. ESMO Open 2022;7:100527. [Crossref] [PubMed]
- Zhou C, Kim SW, Reungwetwattana T, et al. Alectinib versus crizotinib in untreated Asian patients with anaplastic lymphoma kinase-positive non-small-cell lung cancer (ALESIA): a randomised phase 3 study. Lancet Respir Med 2019;7:437-46. [Crossref] [PubMed]
- Zhou C, Lu Y, Kim SW, et al. LBA11 Alectinib (ALC) vs crizotinib (CRZ) in Asian patients (pts) with treatment-naïve advanced ALK+ non-small cell lung cancer (NSCLC): 5-year update from the phase III ALESIA study. Ann Oncol 2022;33:S1563. [Crossref]
- Camidge DR, Kim HR, Ahn MJ, et al. Brigatinib versus Crizotinib in ALK-Positive Non-Small-Cell Lung Cancer. N Engl J Med 2018;379:2027-39. [Crossref] [PubMed]
- Camidge DR, Kim HR, Ahn MJ, et al. Brigatinib Versus Crizotinib in ALK Inhibitor-Naive Advanced ALK-Positive NSCLC: Final Results of Phase 3 ALTA-1L Trial. J Thorac Oncol 2021;16:2091-108. [Crossref] [PubMed]
- Shaw AT, Bauer TM, de Marinis F, et al. First-Line Lorlatinib or Crizotinib in Advanced ALK-Positive Lung Cancer. N Engl J Med 2020;383:2018-29. [Crossref] [PubMed]
- Solomon BJ, Bauer TM, Ignatius Ou SH, et al. Post Hoc Analysis of Lorlatinib Intracranial Efficacy and Safety in Patients With ALK-Positive Advanced Non-Small-Cell Lung Cancer From the Phase III CROWN Study. J Clin Oncol 2022;40:3593-602. [Crossref] [PubMed]
- Cho BC, Kim DW, Bearz A, et al. ASCEND-8: A Randomized Phase 1 Study of Ceritinib, 450 mg or 600 mg, Taken with a Low-Fat Meal versus 750 mg in Fasted State in Patients with Anaplastic Lymphoma Kinase (ALK)-Rearranged Metastatic Non-Small Cell Lung Cancer (NSCLC). J Thorac Oncol 2017;12:1357-67. [Crossref] [PubMed]
- Horn L, Wang Z, Wu G, et al. Ensartinib vs Crizotinib for Patients With Anaplastic Lymphoma Kinase-Positive Non-Small Cell Lung Cancer: A Randomized Clinical Trial. JAMA Oncol 2021;7:1617-25. [Crossref] [PubMed]
- Bearz A, Martini JF, Jassem J, et al. Efficacy of Lorlatinib in Treatment-Naive Patients With ALK-Positive Advanced NSCLC in Relation to EML4::ALK Variant Type and ALK With or Without TP53 Mutations. J Thorac Oncol 2023;18:1581-93. [Crossref] [PubMed]
- Lara-Mejía L, Cardona AF, Mas L, et al. Impact of Concurrent Genomic Alterations on Clinical Outcomes in Patients With ALK-Rearranged NSCLC. J Thorac Oncol 2024;19:119-29. [Crossref] [PubMed]
- Gadgeel S, Peters S, Mok T, et al. Alectinib versus crizotinib in treatment-naive anaplastic lymphoma kinase-positive (ALK+) non-small-cell lung cancer: CNS efficacy results from the ALEX study. Ann Oncol 2018;29:2214-22. [Crossref] [PubMed]
- Camidge DR, Kim HR, Ahn MJ, et al. Brigatinib Versus Crizotinib in Advanced ALK Inhibitor-Naive ALK-Positive Non-Small Cell Lung Cancer: Second Interim Analysis of the Phase III ALTA-1L Trial. J Clin Oncol 2020;38:3592-603. [Crossref] [PubMed]
- Solomon BJ, Bauer TM, Mok TSK, et al. Efficacy and safety of first-line lorlatinib versus crizotinib in patients with advanced, ALK-positive non-small-cell lung cancer: updated analysis of data from the phase 3, randomised, open-label CROWN study. Lancet Respir Med 2023;11:354-66. [Crossref] [PubMed]
- Dziadziuszko R, Peters S, Ruf T, et al. Clinical experience and management of adverse events in patients with advanced ALK-positive non-small-cell lung cancer receiving alectinib. ESMO Open 2022;7:100612. [Crossref] [PubMed]
- Nagasaka M, Ge Y, Sukari A, et al. A user's guide to lorlatinib. Crit Rev Oncol Hematol. 2020;151:102969. [Crossref] [PubMed]
- Reed M, Rosales AS, Chioda MD, et al. Consensus Recommendations for Management and Counseling of Adverse Events Associated With Lorlatinib: A Guide for Healthcare Practitioners. Adv Ther 2020;37:3019-30. [Crossref] [PubMed]
- Zhou Y, Yin Y, Xu J, et al. An update on Alectinib: a first line treatment for ALK-positive advanced lung cancer. Expert Opin Pharmacother 2023;24:1361-73. [Crossref] [PubMed]
- Hubbeling H, Choudhury N, Flynn J, et al. Outcomes With Local Therapy and Tyrosine Kinase Inhibition in Patients With ALK/ROS1/RET-Rearranged Lung Cancers. JCO Precis Oncol 2022;6:e2200024. [Crossref] [PubMed]
- Stinchcombe TE, Doebele RC, Wang X, et al. Preliminary Clinical and Molecular Analysis Results From a Single-Arm Phase 2 Trial of Brigatinib in Patients With Disease Progression After Next-Generation ALK Tyrosine Kinase Inhibitors in Advanced ALK+ NSCLC. J Thorac Oncol 2021;16:156-61. [Crossref] [PubMed]
- Ou SI, Nishio M, Ahn MJ, et al. Efficacy of Brigatinib in Patients With Advanced ALK-Positive NSCLC Who Progressed on Alectinib or Ceritinib: ALK in Lung Cancer Trial of brigAtinib-2 (ALTA-2). J Thorac Oncol 2022;17:1404-14. [Crossref] [PubMed]
- Felip E, Shaw AT, Bearz A, et al. Intracranial and extracranial efficacy of lorlatinib in patients with ALK-positive non-small-cell lung cancer previously treated with second-generation ALK TKIs. Ann Oncol 2021;32:620-30. [Crossref] [PubMed]
- Goto Y, Kenmotsu H, Tamiya M, et al. A Retrospective, Multicenter, Observational Study to Evaluate Clinical Outcomes of Lorlatinib After Alectinib in Patients With ALK-Positive NSCLC in Japan. JTO Clin Res Rep 2023;4:100508. [Crossref] [PubMed]
- Bauer TM, Shaw AT, Johnson ML, et al. Brain Penetration of Lorlatinib: Cumulative Incidences of CNS and Non-CNS Progression with Lorlatinib in Patients with Previously Treated ALK-Positive Non-Small-Cell Lung Cancer. Target Oncol 2020;15:55-65. [Crossref] [PubMed]
- Dagogo-Jack I, Oxnard GR, Evangelist M, et al. Phase II Study of Lorlatinib in Patients With Anaplastic Lymphoma Kinase-Positive Lung Cancer and CNS-Specific Relapse. JCO Precis Oncol 2022;6:e2100522. [Crossref] [PubMed]
- Lin JJ, Schoenfeld AJ, Zhu VW, et al. Efficacy of Platinum/Pemetrexed Combination Chemotherapy in ALK-Positive NSCLC Refractory to Second-Generation ALK Inhibitors. J Thorac Oncol 2020;15:258-65. [Crossref] [PubMed]
- Metro G, Baglivo S, Metelli N, et al. Lorlatinib beyond progression plus platinum/pemetrexed for ALK-positive non-small cell lung cancer patients: report of two cases. J Chemother 2023;35:576-82. [Crossref] [PubMed]
- Guaitoli G, Tiseo M, Di Maio M, et al. Immune checkpoint inhibitors in oncogene-addicted non-small cell lung cancer: a systematic review and meta-analysis. Transl Lung Cancer Res 2021;10:2890-916. [Crossref] [PubMed]
- Nogami N, Barlesi F, Socinski MA, et al. IMpower150 Final Exploratory Analyses for Atezolizumab Plus Bevacizumab and Chemotherapy in Key NSCLC Patient Subgroups With EGFR Mutations or Metastases in the Liver or Brain. J Thorac Oncol 2022;17:309-23. [Crossref] [PubMed]
- Socinski MA, Jotte RM, Cappuzzo F, et al. Atezolizumab for First-Line Treatment of Metastatic Nonsquamous NSCLC. N Engl J Med 2018;378:2288-301. [Crossref] [PubMed]
- Bylicki O, Tomasini P, Radj G, et al. Atezolizumab with or without bevacizumab and platinum-pemetrexed in patients with stage IIIB/IV non-squamous non-small cell lung cancer with EGFR mutation, ALK rearrangement or ROS1 fusion progressing after targeted therapies: A multicentre phase II open-label non-randomised study GFPC 06-2018. Eur J Cancer 2023;183:38-48. [Crossref] [PubMed]
- Ou SI, Nagasaka M, Brazel D, Hou Y, Zhu VW. Will the clinical development of 4th-generation "double mutant active" ALK TKIs (TPX-0131 and NVL-655) change the future treatment paradigm of ALK+ NSCLC?. Transl Oncol 2021;14:101191. [Crossref] [PubMed]
- Wu YL, Tsuboi M, He J, et al. Osimertinib in Resected EGFR-Mutated Non-Small-Cell Lung Cancer. N Engl J Med 2020;383:1711-23. [Crossref] [PubMed]
- Herbst RS, Wu YL, John T, et al. Adjuvant Osimertinib for Resected EGFR-Mutated Stage IB-IIIA Non-Small-Cell Lung Cancer: Updated Results From the Phase III Randomized ADAURA Trial. J Clin Oncol 2023;41:1830-40. [Crossref] [PubMed]
- Leonetti A, Minari R, Boni L, et al. Phase II, Open-label, Single-arm, Multicenter Study to Assess the Activity and Safety of Alectinib as Neoadjuvant Treatment in Surgically Resectable Stage III ALK-positive NSCLC: ALNEO Trial. Clin Lung Cancer 2021;22:473-7. [Crossref] [PubMed]
- Solomon BJ, Ahn JS, Dziadziuszko R, et al. LBA2 ALINA: Efficacy and safety of adjuvant alectinib versus chemotherapy in patients with early-stage ALK+ non-small cell lung cancer (NSCLC). Ann Oncol 2023;34:S1295-6. [Crossref]
- Aredo JV, Mambetsariev I, Hellyer JA, et al. Durvalumab for Stage III EGFR-Mutated NSCLC After Definitive Chemoradiotherapy. J Thorac Oncol 2021;16:1030-41. [Crossref] [PubMed]
- Naidoo J, Antonia S, Wu YL, et al. Brief Report: Durvalumab After Chemoradiotherapy in Unresectable Stage III EGFR-Mutant NSCLC: A Post Hoc Subgroup Analysis From PACIFIC. J Thorac Oncol 2023;18:657-63. [Crossref] [PubMed]
Cite this article as: Facchinetti F, Gandolfi L, Vasseur D, Melocchi L, Nakazawa S, Tiseo M, Friboulet L, Rossi G. ALK fusions turn sixteen in lung cancer: a review on their biology, detection and therapy. Precis Cancer Med 2024;7:2.