A narrative review of biomarkers in advanced triple negative breast cancer
Introduction
Triple negative breast cancer (TNBC) is clinically defined by the lack of expression of the estrogen receptor (ER), progesterone receptor (PR) and low expression of human epidermal growth factor receptor (HER2). As these cancers are defined by what they are not rather than what they are, they naturally represent a heterogenous group of cancers that are still largely managed as a single entity disease.
TNBCs represent 15–20% of breast cancers, are more common in younger women and those of African American descent (1,2), as well as, in BRCA mutation carriers (3). Women with TNBC tend to present with large tumors that are usually higher grade and involve the lymph nodes (4). TNBCs have been characterized by an aggressive natural history with higher rates of relapse within the first 5 years, in addition to higher rates of distant recurrences, worse disease-free survival (DFS) and overall survival (OS) compared to other breast cancer subtypes (4). Despite molecular advances in characterizing TNBCs and the availability of few targeted therapies in the advanced setting, the overall survival of women with metastatic TNBC remains low (4).
TNBCs have been characterized at the genetic and epigenetic levels (5-8), yet therapeutic targets have been lagging. Chemotherapy has been the backbone line of treatment for TNBCs. Recent advances in treatment include immune checkpoint inhibitors (ICIs), such as Atezolizumab with nab-paclitaxel or Pembrolizumab in combination with chemotherapy for PD-L1 positive TNBCs in the metastatic setting (9,10), and PARP inhibitors for previously treated BRCA mutation carriers with metastatic TNBCs (11-13). Antibody drug conjugate (ADC), sacituzumab govitecan, has recently been FDA approved in patients with metastatic TNBC who received at least two prior therapies (14,15). Current challenges include translating the heterogeneity within TNBC to individualized treatment plans for the patient, identifying and utilizing biomarkers that predict survival and/or treatment response and identifying optimal tools to help guide precision medicine. This is in addition to a need to better understand mechanisms of chemoresistance in TNBC. The landscape of biomarker driven targeted therapy in TNBC is rapidly changing, and there are several ongoing clinical trials with potential to personalize the standard care of treatment for this heterogenous disease. Here, we present a review of the recent literature and our current knowledge of the molecular characteristics of this unique subset of breast cancer. Furthermore, we highlight clinically relevant biomarkers that have been described for TNBC, and we focus on emerging potential therapeutic targets. We present the following article in accordance with the Narrative Review Reporting Checklist (available at: http://dx.doi.org/10.21037/pcm-20-76).
Methods
A literature search was conducted on PubMed using the terms ‘advanced triple negative breast cancer’ and ‘biomarkers’ from 2000 to December 2020. The same search terms were used for the ClinicalTrials.gov registry of clinical trials. Abstracts from the annual meetings for the American Society of Clinical Oncology (ASCO), European Society for Medical Oncology (ESMO) and San Antonio Breast Cancer Symposium (SABCS) were included. Only English studies were included.
On the origins of TNBCs: more than basal-like entity
The human breast is organized into a branching system of ducts and lobules. At the cellular level, the breast epithelium is organized into an inner layer of luminal epithelial and an outer layer of basal/myoepithelial cells embedded in a stromal matrix. Each of these cells is characterized by distinct set of genetic features in addition to expression of differentiation markers and show different repopulating capacity in vitro and in vivo (16-18). At the histological level, while luminal epithelial cells stain positive for cytokeratins 8/18, basal epithelial cells stain positive for cytokeratins 5/6, 17 (5,19). Dissection of mammary epithelium in vitro reveals four subpopulations based on cell surface markers, EpCAM and CD49f which are mature luminal cells, luminal progenitor cells, stromal and basal/mammary stem cells. There is data to suggest BRCA1 associated TNBCs which tend to be of the basal-like subtype at the gene expression level arise from the aberrant luminal progenitor subpopulation (16), raising the interest in characterizing mammary stem/progenitor cells to identify potentially actionable targets.
Decrypting the heterogeneity of TNBCs
Immunohistochemical (IHC), DNA, epigenome, RNA, protein and recently immunome analysis have revealed the extensive heterogeneity of TNBCs. While TNBC is defined at the histological level by lack of expression of ER, PR and low expression of HER2, at the molecular level, it is more complex. Initial efforts to molecularly characterize TNBCs from 2 decades ago were through gene expression, revealing TNBCs encompass basal subtype (50–70%), in addition to claudin-low, luminal, HER2+ and normal breast-like subtypes, albeit at lower rates compared to basal subtype (5,6,19-23). Cancers of the basal subtype express basal epithelial markers and tend to occur in younger patients, have poor baseline prognosis, higher mutation rate, higher frequency of TP53 nonsense and frameshift mutations, RB1 and BRCA1 mutations (4,7).
There have been several studies that further examined TNBC at the transcriptional level, subclassifying it into multiple subtypes (24-26). Lehmann et al. (24) initially subclassified TNBC into several subtypes: basal-like (BL1 and BL2), immunomodulatory (IM), mesenchymal (M), mesenchymal stem-like (MSL), luminal androgen receptor (LAR), and unstable (UNS). A subsequent refined analysis which made use of laser-capture microdissection to isolate and specifically characterize tumor cells outside of the tumor microenvironment classified these TNBCs into 4 main subtypes including basal-like (BL1 and BL2), mesenchymal subtype (M), and luminal androgen receptor subtype (LAR) (25). These subtypes have distinct genomic signatures, prognosis and response to chemotherapy (24,27-29).
The BL1 subtype is highly proliferative as determined by elevated expression of proliferation marker Ki67 and is enriched in cell cycle and DNA damage response pathways. The BL2 subtype is enriched in growth factor signalling, glycolysis and gluconeogenesis and expression of myoepithelial markers including TP63. The mesenchymal subtype is defined by its high expression of genes associated with motility and epithelial to mesenchymal transition (EMT). The LAR subtype represents the luminal phenotype and is composed of genes involved in steroid synthesis including androgen receptor, as well as mutations in PIK3CA (24,25).
These subtypes also have distinct clinical characteristics where LAR subtype tends to have more regional nodal involvement, preferentially metastasize to the bone and has a favorable prognosis whereas mesenchymal subtype tends to spread to the lungs and has worse prognosis (25,29). When examining the response to neoadjuvant chemotherapy in TNBCs, the basal subtype had a significantly higher pathological complete response (pCR) compared with mesenchymal subtype while LAR subtype had lowest pCR (30). Burstein et al. used gene expression profiling of 198 TNBCs and identified 4 subtypes: luminal androgen receptor (LAR), mesenchymal (MES), basal-like immunosuppressed (BLIS) and basal-like immune activated (BLIA) (31). BLIS had the worst prognosis whereas BLIA had the best prognosis (31). Similarly, in a transcriptomic analysis of 465 TNBCs by Jiang et al., four subtypes were identified: LAR subtype with enrichment for ERBB2 and PI3K pathway mutations, immunomodulatory subtype, BLIS subtype characterized by upregulation of cell cycle and activation of DNA repair, and a mesenchymal-like subtype enriched in mammary stem cell pathways (26). Overall, gene expression analysis studies showed that classification of TNBC into four subtypes remains unchanged (32). Understanding the biology of these molecular subtypes has helped inform potential subtype targeted therapies. While platinum-based chemotherapy and PARP inhibitors have been suggested to target BL1 subtype and BRCA1 mutation carriers, AR antagonists, PI3K and CDK4/6 inhibitors are suggested to target LAR subtype, and FGFR and NOTCH inhibitors to target M subtype (24-27,29).
The Cancer Genome Atlas (TCGA) Network provided great insight into the heterogeneity of TNBCs through analysis of genomic DNA copy number arrays, DNA methylation, exome sequencing, messenger RNA arrays, microRNA sequence and protein arrays in 510 breast tumors (7). This study confirmed genes already implicated in breast cancer (PIK3CA, PTEN, AKT1, TP53, GATA3, CDH1, RB1, MLL3, MAP3K1 and CDKN1B), as well as provided novel alterations, mutational frequency, genomic alterations commonly observed in luminal A, luminal B, HER2 positive and basal-like subtypes. In this study, 80% of basal-like subtype consisted of TNBCs characterized by mutated TP53 (84%), PIK3CA mutations (7%), PTEN mutation/loss (35%), RB1 mutation/loss (20%), high proliferation, hypomethylation and high expression of DNA repair proteins (7). This data along with the breadth of molecular alterations in breast cancer and TNBCs can be explored through platforms, such as cBioPortal (33).
At a single cell level, treatment naive TNBC exhibits a spectrum of heterogeneity related to mutational burden and clonal evolution; some tumors have high mutational burden whereas other tumors have few somatic mutations. As previously stated TP53 is the most frequently mutated gene. In addition, mutations in PIK3CA, RB1, PTEN, MYO3A and GH1, BRAF V600E, EGFR amplifications and ERBB2/ERBB3 mutations show evidence of single gene selection (8). Commonly activated pathways include TP53 and DNA damage pathway, PIK3 signaling, ERBB2 signaling, integrin signaling and focal adhesion, WNT/cadherin signaling, growth hormone and nuclear receptor co-activators. The TP53 and PIK3CA pathways have higher clonal frequencies, suggesting early role in tumorigenesis (8). The heterogeneity of TNBCs whether at the subtype level or in terms of single cells presents a challenge to identifying potential targets for treatment.
Biomarker targeted therapy in TNBCs
We have reviewed the characteristic features of TNBC subtypes, and in this section, we outline subtype driven biomarkers as potential targets of personalized therapy.
TP53: time to target the guardian
While historically considered a challenge to target tumor suppressor genes, there might be a future for targeting TP53 in TNBC. TP53 is the most frequently mutated gene across various cancers, including breast cancer. TP53 controls functions essential to cell cycle progression, DNA damage repair and apoptosis (34). Among breast cancers subtypes, TP53 is the most frequently mutated gene in TNBCs. TCGA identified 80% of basal subtype cancers were TNBCs with 84% having TP53 mutations (Network, 2012). Clinical and experimental data suggest TP53 mutations constitute an early event in TNBC but not necessarily the first step (7,8,35,36).
TP53 expression is a prognostic marker in TNBC. TP53 IHC expression occurs in 55–70% of TNBC cases and correlates with worse prognosis (37-41). TP53 expression coincided with proliferative TNBCs as determined by high Ki67 expression (41). Overall, TP53 positivity was associated with worse prognosis in TNBC (38,40-42); however, survival was significantly improved with chemotherapy (38). TP53 expression by IHC in TNBC, however, could not predict the response to neoadjuvant chemotherapy (39).
Until Recently, TP53 has proved to be a challenging actionable target; however, compounds that restore wildtype TP53 function to induce cell cycle arrest or apoptosis have been developed. APR-246 is a compound that reactivates mutant TP53 and converts it to a wild type form which has been shown to have anticancer activity in TP53 mutant breast cancer cell lines (43). Another compound, COTI-2, reactivates p53, has shown antitumor activity in TNBC cell lines and is being evaluated in clinical trials (Table 1) (44).
Table 1
Agent | Pathway | Setting | Phase | Sample size | Duration | Identifier |
---|---|---|---|---|---|---|
APR-246 + Pembrolizumab | TP53 + anti-PD-1 | Advanced solid tumors | phase I/II | 118 | Jun 2022 | NCT04383938 |
TNBC, triple negative breast cancer; PD-1, programmed death receptor-1.
Androgen receptor: target hormonally sensitive subset of TNBC
The androgen receptor is a member of the nuclear steroid hormone receptor family which includes ER and PR. Androgens, including testosterone and dihydrotestosterone, activate AR leading to its translocation to the nucleus and concomitant binding to target genes, resulting in downstream transcriptional activation. AR is expressed in >75% of ER positive breast cancer (45,46). AR expression is positive in around 28% of TNBCs (47). There has been mixed data on AR expression and prognostic relevance in TNBC (45-47). A recent systematic review of 27 studies including 4914 patients with TNBC found AR protein expression was not associated with prognosis (47).
AR antagonists have been explored in AR positive metastatic TNBC. In single arm phase 2 clinical trials, bicalutamide use resulted in a six-month clinical benefit rate of 19% and a median PFS of 12 weeks (48). Abiraterone/prednisone demonstrated a 6-month clinical benefit rate of 20% and a median PFS of 2.8 months (49), but it didn’t meet the hypothesis cut-off of clinical benefit of 25%. AR positivity in these studies was determined by protein expression >10% by IHC. The Enzalutamide study included patients with AR positive status defined as AR expression >0% and revealed a 16-week clinical benefit rate of 25%, median PFS of 2.9 months and a median OS of 12.5 months (50). Interestingly, patients with AR expression >10% had a 16-week clinical benefit rate of 33%, median PFS of 3.3 months and a median OS of 16.5 months suggesting an AR expression level dependent response; however, based on the study, AR expression by IHC was suboptimal as a predictive biomarker. Overall, the data suggests targeting AR might be beneficial in a selective niche of patients with metastatic TNBC and positive AR expression and warrants further study. Alternatively, combined targeting of AR and activated downstream signaling pathways are proposed to be more effective in this subgroup. Given that the LAR subtype has frequent PIK3CA mutations, there is potential to combine PI3K inhibition and AR antagonism in AR positive TNBC. Ongoing Phase I/II clinical trials are investigating enzalutamide plus alpelisib or taselisib, PI3K inhibitors, in patients with AR positive TNBCs (Table 2). In vitro and in vivo studies using LAR TNBC cell lines showed high sensitivity to CDK4/6 inhibitor (51). Accordingly, there is an ongoing clinical trial looking at combining ribociclib with bicalutamide in metastatic AR positive TNBCs (Table 2).
Table 2
Agent | Targets of therapy | Setting | Phase | Sample size | Completion date | Identifier |
---|---|---|---|---|---|---|
Ribociclib + bicalutamide | CDK4/6 + NSAA | Metastatic | Phase I/II | 11 | Sept 2021 | NCT03090165 |
Bicalutamide | NSAA | Metastatic | Phase III | 262 | Dec 2020 | NCT03055312 |
Enzalutamide | NSAA | Metastatic | Phase II | 118 | Mar 2021 | NCT01889238 |
Darolutamide verses capecitabine | NSAA versus chemotherapy | Metastatic | Phase II | 90 | Sept 2021 | NCT03383679 |
Taselisib + enzalutamide | NSAA + PI3Ki | Metastatic | Phase I/II | 30 | Dec 2020 | NCT02457910 |
Alpelisib + enzalutamide | NSAA + PI3Ki | Metastatic | Phase I | 28 | Dec 2020 | NCT03207529 |
Pembrolizumab + enobosarm | Anti-PD-1 + SARM | Metastatic | Phase II | 29 | Nov 2020 | NCT02971761 |
CR1447 (4-OH-testosterone) | Steroidal AI | Metastatic | Phase II | 90 | Jun 2027 | NCT02067741 |
Orteronel | Nonsteroidal CYP17A1 inhibitor | Metastatic | phase II | 71 | Dec 2020 | NCT01990209 |
TNBC, triple negative breast cancer; NSAA, nonsteroidal antiandrogen; AR, androgen receptor; SARM, selective androgen receptor modulator; PI3Ki, PI3K inhibitor; AI, aromatase inhibitor.
BRCA: the beginning to a world of targeting synthetic lethality
BRCA1 is a tumor suppressor gene involved in DNA damage repair of double strand breaks through homologous recombination (52). Women who inherit germline mutations in BRCA1/ BRCA2 have increased risk of developing breast (50–80%) and ovarian cancer (40% vs. 20%, respectively) (53-55), with BRCA2 carries likely at a higher risk of breast cancer (53,56). BRCA1 mutation carriers predominantly develop basal subtype while BRCA2 mutations are mostly associated with luminal B subtype (57-59). Germline mutations in BRCA genes are present in 10% of TNBC (55).
As a biomarker, BRCA1/2 mutation status may influence treatment selection. Loss of BRCA results in homologous recombination deficiency (HRD) rendering cells sensitive to platinum chemotherapy as well as to inhibitors of the DNA repair enzyme poly-ADP ribose polymerase (PARP). In the TNT Trial, a phase III study in advanced TNBC patients, BRCA mutation carriers treated with carboplatin had a 2-fold increase in overall response rate as compared to those treated with docetaxel. This benefit was limited to BRCA1 mutation carriers and was not observed in tumors with HRD or BRCA1 methylation (60). Interestingly, the location of BRCA mutation may impact the level of HRD and sensitivity to platinum as well as PARP inhibitors in TNBC (61-63).
PARP inhibitors use the concept of synthetic lethality resulting from the inability of BRCA deficient cells to repair double strand breaks (DSBs) (64). PARP use base excision repair (BER) to repair DNA damage at the site of single strand breaks (SSBs). Inhibiting PARP results in accumulation of SSBs which stall the replication fork causing DSBs. In the absence of functional BRCA, DSBs accumulate causing cell death (64-66). Three major clinical trials showed efficacy of PARP inhibitors in metastatic TNBC in germline BRCA mutation carriers including phase III OLYMPIAD, EMBRACA, BROCADE3 (11,12,67). In OlympiAD, patients with BRCA1/2 germline alteration were randomly assigned to receive olaparib or standard therapy with single-agent chemotherapy of physician’s choice. Median PFS was significantly longer in the olaparib group than in the standard-therapy group (7.0 vs. 4.2 months, respectively). The response rate was 59.9% in the olaparib group and 28.8% in the control group. In EMBRACA, patients with advanced breast cancer and a germline BRCA1/2 mutation were assigned to receive talazoparib or standard single-agent therapy of physician’s choice (11). Median PFS was significantly improved in the talazoparib group than in the standard-therapy group (8.6 vs. 5.6 months, respectively) (11). The objective response rate was higher in the talazoparib group than in the standard-therapy group (62.6% vs. 27.2%) (11). In the BROCADE3 trial, adding veliparib to platinum doublet, with continuation as monotherapy if the doublet were discontinued, resulted in significant improvement in PFS in BRCA patients with advanced TNBCs (14.5 vs. 12.6 months) (67). Up to this point, PARP inhibitors did not show a statistically significant overall survival (OS) benefit for patients with metastatic breast cancer and mutations in the BRCA1/2 genes. Interestingly, PARP inhibitors may modulate the tumor microenvironment which may improve response to immunotherapy. As such, there are ongoing clinical trials investigating combined PARP inhibition and immunotherapy (Table 3) (68).
Table 3
Agent | Targets of therapy | Setting | Phase | Sample size | Completion Date | Identifier |
---|---|---|---|---|---|---|
Olaparib + carboplatin/paclitaxel | PARPi + chemotherapy | Metastatic | I | 189 | Dec 2020 | NCT00516724 |
MEDI4736 + olaparib and/or cediranib | Anti-PD-L1 + PARPi + anti-VEGFR | Advanced | I/II | 384 | Dec 2022 | NCT02484404 |
Olaparib + durvalumab | PARPi + anti-PD-L1 | Metastatic | Phase II | 28 | Dec 2020 | NCT03801369 |
Veliparib + cisplatin | PARPi + chemotherapy | Metastatic | Phase II | 333 | Oct 2021 | NCT02595905 |
Talazoparib | PARPi | Recurrent/metastatic (no requirement for BRCA mutation) | Phase II | 49 | Jan 2022 | NCT03901469 |
Talazoparib | PARPi | Metastatic | Phase II | 40 | Dec 2022 | NCT02401347 |
Olaparib + onalespib | PARPi + anti-HSP90 | Recurrent/refractory/metastatic | Phase I | 40 | Dec 2020 | NCT02898207 |
BKM120/BYL719 + olaparib | PI3Ki + PARPi | Recurrent/metastatic | Phase I | 118 | Dec 2020 | NCT01623349 |
PARPi, poly ADP ribose polymerase inhibitor; TNBC, triple negative breast cancer; PD-L1, programmed death ligand-1; VEGFR, vascular endothelial growth factor receptor; HSP90, heat shock protein 90.
Homologous recombination deficiency (HRD): targeting HRD beyond BRCA
Homologous recombination (HR) is essential for genomic integrity. While the previously described mutations in BRCA1/BRCA2 highlighted the connections between homologous recombination deficiency (HRD) and cancer predisposition, the term HRD is a broader entity that encompasses various mechanisms contributing to a “BRCAness” phenotype (64). This includes genetic inactivation of other components of the HR pathway at the germline or somatic level including PALB2, BARD1, BRIP1, RAD51B, RAD51C, RAD51D, ATM, CHEK2 or mutations in non-HR gene mutations, such as MSH6 and PTEN or epigenetic hypermethylation of BRCA1. The BRCAness feature is predictive of response to platinum-based chemotherapy and PARP inhibitors. Identifying assays that can predict BRCAness phenotype and corresponding response to platinum/PARP inhibitors has been the focus of several research studies. Genomic signatures, RAD51 foci and HRD scores have been considered as potential assays (69-72).
Genomic signatures have been previously described in breast, ovarian and pancreatic patients with BRCA1/2 mutations (69). Signature 3, a signature characterized by tandem genomic duplications, has been shown to be associated with HRD, BRCA1/2 and PALB2 mutations as well as RAD51C promotor methylation but not ATM or CHEK2 mutations (73). Another assay is the HRD score, the sum of three metrics of chromosomal level aberration including loss of heterozygosity (LOH), telomeric allelic imbalance (TIA), and large-scale state transitions (LST). HRD score >42 and/or tumor BRCA1/2 mutation identified tumors that respond to neoadjuvant platinum-containing chemotherapy in patients with TNBC (74). In a study that incorporated HRD score to identify BRCAness, the addition of velaparib to cisplatin significantly improved PFS and showed a trend towards improved OS for BRCA-like advanced TNBC (74). In addition, olaparib was shown to have activity in metastatic breast cancer patients with somatic BRCA1/2 alterations and germline PALB2 but not in patients with ATM and CHEK2 alterations (75). BRCA1 promoter hypermethylation represents another form of BRCAness. In an analysis of 237 early TNBC cases, 24.1% of TNBC patients were BRCA1 hypermethylated which is more frequent than BRCA mutations (70). A higher frequency of BRCA1 hypermethylation was noted in young patients and was associated with basal phenotype. However, in the TNT trial, BRCA1 hypermethylation was not associated with response to platinum-based therapy (60). Identifying an optimal test that captures the BRCAness phenotype is the focus of ongoing research, as well as exploring the use of PARP inhibitors in metastatic TNBCs with alterations in the HR pathway (Table 4).
Table 4
Agent | Targets of therapy | Setting | Phase | Sample size | Completion Date | Identifier |
---|---|---|---|---|---|---|
HX008 + niraparib | Anti-PD-1 + PARPi | Metastatic | Phase II | 44 | Apr 2022 | NCT04508803 |
AMXI-5001 | Dual PARPi and microtubule polymerization inhibitor | Metastatic/advanced | Phase 1/II | 80 | Jan 2023 | NCT04503265 |
Prexasertib | CHK1 inhibitor | Advanced | Phase II | 50 | Apr 2022 | NCT02873975 |
Talazoparib | PARPi | Advanced | Phase II | 40 | Dec 2022 | NCT02401347 |
Olaparib | PARPi | Metastatic | Phase II | 39 | Nov 2021 | NCT03367689 |
High dose chemotherapy (carboplatin, thiotepa, and cyclophosphamide) | Chemotherapy | Metastatic | Phase III | 74 | Oct 2023 | NCT01646034 |
IDX-1197 | PARPi | Metastatic | Phase I/II | 310 | Mar 2023 | NCT04174716 |
Olaparib + pembrolizumab | PARPi + anti-PD-1 | Metastatic/advanced | Phase II | 300 | Dec 2023 | NCT04123366 |
Niraparib + carboplatin | PARPi + chemotherapy | Advanced | Phase I | 146 | July 2022 | NCT03209401 |
Olaparib | PARPi | Metastatic | Phase II | 390 | Feb 2024 | NCT03742895 |
HRD, homologous recombination deficiency; TNBC, triple negative breast cancer; PARPi, poly ADP ribose polymerase inhibitor; PD-L1, programmed death ligand-1.
PI3K/AKT/MTOR pathway: time to tackle kinase world
The PI3K/AKT/mTOR kinases regulate key pathways essential to cell survival, proliferation and differentiation and are activated through different mechanisms in TNBC (76). PIK3CA mutations are associated with luminal cancers and the LAR subtype of TNBCs (7,26). In basal-like cancers, PI3K/AKT pathway activation is mediated through a different mechanism, i.e., loss of negative regulators of the PI3K pathway such as PTEN and INPP4B phosphatase (7,77,78). PTEN protein expression loss by IHC is significantly associated with large tumor size, high grade, recurrence and TNBC, as well as poorer prognosis (79) while INPP4B loss is associated with higher tumor grade and basal-like breast cancers (77). In mouse models, INPP4B loss led to dose-dependent increase in tumor incidence in INPP4B homozygous and heterozygous knockout mice compared to wild-type mice, supporting a role for INPP4B as a tumor suppressor in TNBC (78). Another mechanism of pathway activation includes mutations in the catalytic subunit of PI3K (p110α) which occur in about 10% of TNBC cases (7). Contrary to hormone receptor positive breast cancer, PIK3CA mutations in TNBCs are associated with improved survival (80).
The LOTUS trial is a phase II clinical trial including patients with treatment native metastatic TNBC who were randomized to paclitaxel plus either ipatasertib, oral ATP-competitive small molecule AKT inhibitor, or placebo. median OS (mOS) was 25.8 months in the ipatasertib plus paclitaxel arm vs. 16.9 months in the placebo plus paclitaxel (81). Interestingly, PTEN-low and PIK3CA/AKT1/PTEN altered subgroups had better OS in ipatasertib plus paclitaxel group (81). This, however, did not translate to a meaningful benefit in phase III IPATunity130 that randomized patients with advanced TNBC and alterations in the PIK3CA/AKT1/PTEN pathway to ipatasertib plus paclitaxel verses placebo plus paclitaxel. There was similar overall response rate between the ipatasertib plus paclitaxel verses placebo plus paclitaxel arms (39% vs. 35%, respectively). At a median follow-up of 8.3 months, PFS was similar between the experimental and placebo arms (7.4 vs. 6.1 months, respectively) (82). This suggests there is likely redundant downstream signaling that bypasses AKT mediated inhibition. This is pending further analysis to explore potential biomarkers of benefit from ipatasertib in this trial. Interestingly, addition of ipatasertib to atezolizumab and chemotherapy (paclitaxel or nab-paclitaxel) in 26 patients with advanced TNBC had an objective response rate of 73% seen regardless of PD-L1 or PIK3CA/AKT/PTEN pathway alteration status (83), suggesting a promising trend toward combining targeted therapies in TNBC.
Another phase II trial, the PAKT trial, investigated capivasertib, an oral AKT inhibitor, with paclitaxel versus paclitaxel alone as first-line treatment of metastatic TNBC. With capivasertib, PFS improved (5.9 vs. 4.2 months, respectively) and in patients with PIK3CA/AKT1/PTEN alterations, this benefit was prominent (PFS, 9.3 vs. 3.7 months, respectively) (84). An improvement in median OS was seen in the entire population (19.1 vs. 12.6 months) (84). A better understanding of the redundancy in the pathway and the main downstream drivers is required to drive precision medicine. Clinical trials are currently ongoing to evaluate PI3K/AKT/mTOR inhibitors in treating TNBC (Table 2).
Immunotherapy and tumor microenvironment: a world of hot and cold and all in-between
The advent of immunotherapy to the realm of metastatic TNBC treatment has triggered interest in identifying biomarkers of response. Compared to other breast cancer subtypes, TNBC has an immune rich tumor microenvironment characterized by tumor infiltrating lymphocytes (TILs), expression of immune markers including programmed death-ligand 1 (PD-L1) and high tumor mutational burden (TMB) (85).
PD-L1 is a cell membrane protein expressed on tumor cells and immune cells (IC). PD-L1 binds to PD-1 on T cells to inhibit their antitumor function (86). Immune checkpoint inhibitors (ICIs), including anti-PD-L1/anti-PD-1 release the inhibition on T cells thereby activating the immune system to attack cancer cells. Currently, PD-L1 is approved as a biomarker for selection of patients who are most likely to benefit from immunotherapy in metastatic TNBC based on findings from the IMpassion130 trial for atezolizumab and nab-paclitaxel use, as well as Keynote-355 for pembrolizumab plus chemotherapy (9,10,87).
In examining the prognostic value of PD-L1 expression by IHC, there are mixed results depending on type of assay, differential expression of PD-L1 on tumor verses immune cells, in addition to differential expression of PD-L1 in early verses metastatic settings as well as differential expression by site of metastases (88). In a systematic review and meta-analysis of PD-L1 expression from five studies, four of which included early-stage breast cancer, encompassing a total of 2,546 breast cancers, Zhang et al. showed PD-L1 positivity is in the range 21–56%. PD-L1 expression was associated with positive lymph node metastasis, higher histological grade, estrogen receptor negativity, and TNBC (89). In another systematic review and meta-analysis of PD-L1 expression in breast cancer, PD-L1 positivity rate was 24% in tumor cells, 33% in immune cells and 25% in both with highest PDL-L1 expression in TNBC. PD-L1 expression in breast tumors was associated with shorter DFS and OS. However, in TNBC subtype, PD-L1 expression in immune cells was associated with improved DFS and OS. In a study examining PD-L1 expression by IHC using SP142 antibody in 223 TNBC cases and assessed in tumor cells (TC) as well as tumor-infiltrating lymphocytes (TILs), PD-L1 expression was detected in both tumor cells and TILs at a level of 8.5% and 25.1% respectively (90). PD-L1 expression on TILs but not tumor cells was associated with a poor outcome (90). Overall, the data suggests PD-L1 expression is higher in TNBC and in the immune cell compartment, and the data for prognosis is heterogeneous. The use of different antibodies has contributed to the complexity.
PDL-1 is predictive of response to immunotherapy based on the clinical trials (Tables 5,6). In IMpassion 130, patients with untreated metastatic TNBC cancer were randomized to receive atezolizumab plus nab-paclitaxel or placebo plus nab-paclitaxel (9,87). Forty percent of the tumors were PDL1 positive. While there was no significant difference in OS between the two groups in the intent-to-treat (ITT) analysis (mOS of 21 months in the atezolizumab plus nab-paclitaxel versus 18.7 months in the placebo plus paclitaxel), in the PD-L1 positive population, median OS was longer with atezolizumab plus nab-paclitaxel versus placebo plus nab-paclitaxel at 25.4 and 17.9 months, (stratified HR 0.71, 0.54–0.94), respectively (9,87). In Keynote-355 trial, patients with untreated metastatic TNBC cancer were randomized to receive pembrolizumab plus chemotherapy versus placebo plus chemotherapy (10). Among 847 patients, 25% had PD-L1 CPS <1, 75% had PD-L1 CPS of ≥1 or more, and 38% had PD-L1 CPS of ≥10 (10). Among patients with CPS ≥10, mPFS was statistically significantly higher in the pembrolizumab plus chemotherapy group compared to placebo plus chemotherapy (9.7 vs. 5.6 months, respectively). mPFS was not significant among patients with CPS of ≥1 (7.6 vs. 5.6 months, respectively). The data indicated treatment response is PD-L1 expression dependant, and OS data is yet to be reported (10).
Table 5
Agent | Targets of therapy | Setting | Phase | Sample size | Completion data | Identifier |
---|---|---|---|---|---|---|
Avelumab | Anti-PD-L1 | Metastatic | Phase II | 620 | Feb 2024 | NCT02554812 |
Atezolizumab + BDB001 + RT | Anti-PD-L1 + TLR agonist | Metastatic | Phase II | 247 | Jan 2025 | NCT03915678 |
Atezolizumab | Anti-PD-L1 | Metastatic | Phase II | 200 | Oct 2024 | NCT04273061 |
Nivolumab + bicalutamide + ipilimumab | Anti-PD-1+ AR antagonist+ anti-CTLA4 | Metastatic | Phase II | 138 | Apr 2025 | NCT03650894 |
Nivolumab + eribulin | Anti-PD-L1 + chemotherapy | Metastatic | Phase I/II | 90 | Sep 2021 | NCT04061863 |
PVX-410 vaccine+ pembrolizumab in HLA-A2 positive patients | Vaccine + anti-PD-1 | Metastatic | Phase I | 20 | Dec 2024 | NCT03362060 |
Pembrolizumab + olaparib | Anti-PD-1 + PARPi | Metastatic | Phase I | 20 | Nov 2025 | NCT03025035 |
Durvalumab + tremelimumab + metronomic vinorelbine | Anti-PD-L1 + anti-CTLA4 + chemotherapy | Metastatic | Phase I/II | 150 | Dec 2024 | NCT03518606 |
Avelumab + binimetinib, utomilumab, or anti-OX40 antibody | Anti-PD-L1 + MEK 1/2 inhibitor, CD 137 agonist or anti-OX40 | Metastatic | Phase II | 150 | July 2021 | NCT03971409 |
Cryoablation + atezolizumab + nab-paclitaxel | Anti-PD-L1 + chemotherapy | Advanced/metastatic | Phase I | 5 | Dec 2020 | NCT04249167 |
Atezolizumab in different combinations | Anti-PD-L1 in different combinations, including chemotherapy, ADC, anti-CD40, anti-IL6R, anti-VEGFA, anti-AKT inhibitor | Metastatic | Phase 1/II | 280 | Jan 2022 | NCT03424005 |
Spartalizumab + LAG525 in combination with NIR178, capmatinib, MCS110, or canakinumab | Anti-PD-1 + anti-LAG-3 in combination with anti- adenosine A2A receptor, anti-Met receptor, anti-CSF-1 or anti-IL1β | Advanced/metastatic | Phase I | 220 | Jan 2022 | NCT03742349 |
PD-L1, programmed death ligand-1; PD-1, programmed death receptor-1; TLR, Toll-like receptor; CTLA4, cytotoxic T-lymphocyte-associated protein 4; MEK1/2, mitogen activated protein kinases; PARPi, poly ADP ribose polymerase inhibitor; ADC, antibody drug conjugate.
Table 6
PD-1/PD-L1 Inhibitors | Phase | Indication | Regimen | PD-L1 Assay | PD-L1 Cuttoff | Overall PD-L1 expression level | Results | References |
---|---|---|---|---|---|---|---|---|
Pembrolizumab, N=32 | Phase 1 | Heavily pretreated | Pembrolizumat 10 mg/kg IV q2 weeks | PD-L1 IHC 22C3pharmDx assay | PD-L1 in the stroma or ≥1% of tumor cells | 111 patients were screened for the trial and 58.6% had positive PD-L1 status | ORR, 18.5% (6.3–38.1%); mPFS, 1.9 (1.7–5.5) months; mOS, 11.2 (5.3–NR) months | Keynote-012 (91) |
Pembrolizumab, N=170 | Phase II | Second line or later line | Pembrolizumab 200 mg IV q3 weeks | PD-L1 IHC 22C3pharmDx assay | CPS ≥1 | 61.8% had PD-L1 positive status | Data analysis on PD-L1 positive vs. overall population; ORR, 5.7% (2.4–12.2%) vs. 5.3% (2.7–9.9%); mPFS, 2.0 (1.9–2.1) vs. 2.0 (1.9–2.0) months; mOS, 8.8 (7.1–11.2) vs. 9.0 (7.6–11.2) months | Keynote-086, cohort A (92) |
Pembrolizumab, N=84 | Phase II | First line | Pembrolizumat 200 mg IV q3 weeks | PD-L1 IHC 22C3pharmDx assay | CPS ≥1 | 61.8% had PD-L1 positive status | ORR, 21.4% (13.9–31.4%); mPFS, 2.1 (2.0–2.2) months; mOS, 18.0 (12.9–23.0) months | Keynote-086, cohort B (93) |
Pembrolizumab verses chemotherapy, N=622 | Phase III | Second line or later line | Pembrolizumab 200 mg IV q3 weeks | PD-L1 IHC 22C3pharmDx assay | CPS ≥1 | 65% had PD-L1 CPS of ≥1, 31% had PD-L1 CPS ≥10, and 18% had PD-L1 CPS ≥20 | Data analysis on PD-L1 CPS ≥10 cases vs. PD-L1 CPS ≥1 case vs. overall population vs. control arm: ORR, 17.7% (10.7–26.8%) vs. 12.3% (8.1–17.6%) vs. 9.6% (6.6–13.4%) vs. 10.6% (7.4–14.6%); mPFS, 2.1 (2.0–2.5) vs. 2.1 (2.0–2.1) vs. 2.1 (2.0–2.1) vs. 3.3 (2.7–4.0) months; mOS, 12.7 (9.9–16.3) vs. 10.7 (9.3–12.5) vs 9.9 (8.3–11.4) vs 10.8 (9.1–12.6) months | Keynote-119(94) |
Atezolizumab + nab-paclitaxel, N=33 | Phase 1 | 0 to 2 lines of prior chemotherapy | Atezolizumab IV 800 mg on days 1 and 15 of each cycle q2 weeks and nab-Paclitaxel, 125 mg/m2, on days 1, 8, and 15 of each cycle (3 weeks on, 1 week off) | Roche Ventana SP142 | Exploratory analysis on PD-L1 IC ≥1 | PD-L1 expression was more frequent on immune cells (50%) compared with tumor cells (17%) | Data analysis on PD-L1 positive cases verses overall population: ORR, 41.7% (15.2–72.3%) vs. 39.4 (22.9–57.9%); mPFS, 6.9 (5.2–11.0) vs. 5.5 (5.1–7.7) months; mOS, 21.9 (13.1–NE) vs. 14.7 (10.1–NE) months | (95) |
Atezolizumab +nab-paclitaxel verses chemotherapy, N=902 | Phase III | First line | Atezolizumab IV 840 mg on days 1 and 15 of each cycle q2 weeks and nab-paclitaxel, 100 mg/m2, on days 1, 8, and 15 of each cycle (3 weeks on, 1 week off) | Roche Ventana SP142 | IC≥1% | 40.9% had PD-L1 positive status | Data analysis on PDL1 IC ≥1 case vs. overall population vs. control arm: ORR, 58.9% (51.5–66.1%) vs. 56% (51.3–60.6%) vs. 45.9% (41.2–50.6%); mPFS, 7.5 (6.7–9.2) vs. 7.2 (5.6–7.5) vs. 5.5 (5.3–5.6) months; mOS, 25 (19.5–30.7) vs. 21 (19–22.6) vs. 18.7 (16.9–20.3) months | IMpassion130 (9,87) |
Atezolizumab + paclitaxel verses placebo plus paclitaxel, N=651 | Phase III | First line | Atezolizumab IV 840 mg on days 1 and 15 of each cycle q2 weeks and paclitaxel, 90 mg/m2, on days 1, 8, and 15 of each cycle (3 weeks on, 1 week off) | Roche Ventana SP142 | IC ≥1% | 44.7% had PD-L1 positive status | Data analysis on PD-L1 IC ≥1 case vs. overall population vs. control arm: ORR, 63.5% vs. 53.6% vs. 47.5%; mPFS, 7.5 (6.7–9.2) vs. 7.2 (5.6–7.5) vs. 5.5 (5.3–5.6) months; mOS, 22.1 vs. 19.2 vs. 22.8 months | IMpassion131 (96) |
Pembrolizumab with chemotherapy verses chemotherapy, N=847 | Phase III | First line | Pembrolizumab 200 mg IV q3 weeks plus chemotherapy (nab-paclitaxel; paclitaxel; or gemcitabine plus carboplatin) or placebo plus chemotherapy | PD-L1 IHC 22C3 pharmDx | CPS ≥1 | 75% had PD-L1 CPS ≥1 and 38% had PD-L1 CPS ≥10 | Data analysis on CPS ≥10 cases vs. CPS ≥1 case vs control arm; interim analysis: mPFS 9.7 vs. 7.6 vs. 5.6 months | Keynote-355 (10) |
ORR, overall response rate; mPFS, median progression free survival; mOS, median overall survival; CPS, composite positive score; PD-L1, programmed death ligand-1; IHC, immunohistochemistry; IC, immune cells.
There is a technical component to assessing PD-L1 expression status in these trials. PD-L1 positivity was defined by PD-L1 expression in immune cells using the Ventana SP142 IHC assay in the IMpassion130 (9,87). Whereas PD-L1 positivity was determined by the combined positive score (CPS) defined as the ratio of PD-L1 expressing cells (tumor cells, lymphocytes and macrophages) to the number of all tumor cells, using the DAKO PD-L1 22C3 IHC assay in Keynote-355 (10). Of note, these assays identified different percentages of PD-L1 positive cases and antibodies are not interchangeable.
Furthermore, genomic analysis of 641 TNBCs identified PD-L1 amplification (3%), TMB of ≥10 mutations/Megabase mut(mb) (9%), MSI‐high (0.4%) in addition to positive PD‐L1 staining of ICs (47%) as potential markers of benefit to ICIs whereas inactivating STK11 mutations (2%) and MDM2 amplification (3%) confer resistance to ICI (85). High levels of TILs appear to have a better prognosis in TNBC patients (97). High TIL expression correlates with PD-L1 in the tumor microenvironment (97). TILs, PD-L1 along with other biomarkers for ICIs, such as TMB, PD-L1 gene expression, neoantigen burden, T-regulatory cells, myeloid-derived suppressor cells, immune signatures, gut microbiome represent complex interactions of the immune system, host and the tumor. In fact, 36 different variables of response to immune checkpoint inhibitors have been identified across tumor types with CD8-T-cells abundance, TMB and high PD-1 gene expression being most predictive of response to anti-PD-1/PD-L1 therapy (98). Therefore, there’s no one size fits all to predict response to immune checkpoint inhibition and likely a tailored approach to patient’s immunome may be necessary.
ADC: time for loaded guns
ADCs are multiagent drugs aimed at tumor targeted delivery of therapeutic small molecules and have shown promising results in TNBC. ADCs include three agents: an antibody directed to a tumor antigen, a cytotoxic molecule, and a linker in between (14). Sacituzumab govitecan is an anti-trophoblast cell-surface antigen (Trop-2) antibody conjugated to a DNA damaging agent, SN-38, via a pH-sensitive cleavable linker. Elevated expression of Trop-2 in breast cancer is correlated with poor prognosis (14). In a single-arm phase I/II study, 108 patients with metastatic TNBC treated with at least two prior therapies received sacituzumab govitecan with objective response rate (ORR) of 33.3%, median PFS of 5.5 months and median OS of 13.0 months regardless of Trop-2 expression in tumors (14). In a phase III trial, the study compared sacituzumab govitecan with single-agent chemotherapy in 468 patients with relapsed/refractory TNBC. Median PFS was significantly longer with sacituzumab govitecan versus control group (5.6 vs. 1.7 months, respectively). Median OS was 12.1 months with sacituzumab govitecan compared to 6.7 months with chemotherapy and objective response rates were 35% and 5%, respectively (15). Side effect profile is similar to other chemotherapy drugs and include but are not limited to neutropenia, anemia, GI symptoms such as nausea, diarrhea as well alopecia and fatigue. Common grade 3 or 4 toxicities included neutropenia, diarrhea, anemia. This led to accelerated approval by FDA for adult patients with metastatic TNBC who had received at least two prior therapies. Another ADC, Ladiratuzumab vedotin, is a humanized antibody targeting the zinc transporter LIV-1 conjugated with a microtubule-disrupting agent, monomethyl auristatin E (MMAE) by a proteolytically cleavable linker. LIV-1 is a multi-span transmembrane protein with putative zinc transporter and metalloproteinase activity expressed in 68% of metastatic TNBC tumors (99). Interim results of the phase I study showed promising clinical activity of ladiratuzumab vedotin with key adverse events including GI symptoms, neutropenia and peripheral neuropathy (100). Trastuzumab deruxtecan, a humanized antibody against HER2 conjugated with a topoisomerase I inhibitor, extecan derivative (DXd) by a cleavable peptide linker, has shown activity in low HER2 (IHC 1+ or 2+/ISH negative) expressing metastatic breast cancer (101) and is currently under further investigation. Ongoing clinical trials with ADCs in metastatic TNBC are outlined in Table 7.
Table 7
Agent | Targets of therapy | Setting | Phase | Sample size | Completion date | Identifier |
---|---|---|---|---|---|---|
SGN-LIV1A (Ladiratuzumab Vedotin) | ADC | Metastatic | Phase I/II | 418 | Mar 2022 | NCT01969643 |
Sacituzumab govitecan + talazoparib | ADC + PARPi | Metastatic | Phase I/II | 65 | Aug 2024 | NCT04039230 |
DS-8201a (Trastuzumab Deruxtecan) | Anti-HER2 ADC | Metastatic | Phase II | 162 | Apr 2026 | NCT04132960 |
ADC, antibody drug conjugate; PARPi, poly ADP ribose polymerase inhibitor; HER2, human epidermal growth factor receptor 2.
Conclusions
While the identification of multiple molecular subtypes of TNBC and the characterization of their unique tumor microenvironments have increased our knowledge and understanding of TNBC, the application of these findings has not yet been adapted in the clinical setting. The median overall survival of metastatic TNBC has improved from 12 months to around 24 months as seen in clinical trials due to the availability of more lines of treatment for this subtype. Although PARP inhibitors and immune checkpoint inhibitors have been recently integrated into the therapeutic arsenal, cytotoxic chemotherapy remains the backbone of therapy of TNBC.
Current research is focused on identifying biomarkers that may potentially serve as therapeutic targets, prognostic markers, or predictors of therapeutic response. Several promising markers have been described, but there still remains a need for further validation in prospective clinical studies. Identifying a gene signature panel that can subclassify TNBCs into their respective subtypes would help personalize therapeutic targets. Meanwhile there is a potential to identify the four common TNBC subtypes classified based on gene expression through various established tests that would help guide management in the metastatic setting (Figure 1). AR testing through IHC and PIK3CA mutational status can classify LAR subtype. This, in turn, would identify a niche of patients who would benefit from AR antagonists alone or in combination with PI3K inhibitor in patients who harbor PIK3CA mutations to overcome the redundancy in the pathway or alternatively CDK4/6 inhibitor. In the immune activated subtype, using PD-L1 status by IHC selects patients who would benefit from immunotherapy with the caveat that PD-L1 antibody tests are not interchangeable and may not be depictive of the true population that would mostly benefit for ICI. Biomarkers of response to ICI under investigation include TILs, TMB and MSI-H. There is also interest in understanding the tumor microenvironment and turning immunologically cold tumors into hot ones that can respond to immunotherapy. For the basal immunosuppressed subtype, characterized by genomic instability, identifying BRCAness phenotype in addition to BRCA1/2 mutations would help inform the response to platinum-based chemotherapy and PARP inhibitors, taking into account that certain mutations, such as ATM and CHEK2 don’t respond to these treatments. Expanding the tests to include somatic BRCA1/2 mutations, BRCA1 methylation status and HRD score have potential for a broader selection of tumors with BRCAness phenotype. For the mesenchymal subtype, there are no current actionable targeted therapies; future studies would look into characterizing cancer stem cells (CSCs) and possible targeting of driver pathways, such as the JAK-STAT pathway. The advent of ADCs has opened a world of multi-drug targeted therapies in heavily treated metastatic TNBC awaiting more combination drugs in clinical trials.
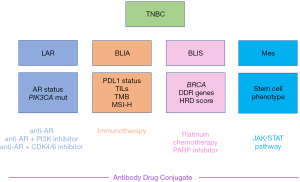
To sum it up, as we head toward more personalized treatments in TNBCs, there is a need to manage the heterogeneity of the disease with finesse; that would require a multi-modal arsenal of biomarker driven targets. More importantly, a uniform and accessible panel of biomarkers is warranted to allow for wider universal adoption.
Acknowledgments
Funding: None.
Footnote
Provenance and Peer Review: This article was commissioned by the Guest Editor (Jacques Raphael) for the series “Management of Triple Negative Breast Cancer” published in Precision Cancer Medicine. The article has undergone external peer review.
Reporting Checklist: The authors have completed the Narrative Review Reporting Checklist. Available at: http://dx.doi.org/10.21037/pcm-20-76
Peer Review File: Available at: http://dx.doi.org/10.21037/pcm-20-76
Conflicts of Interest: All authors have completed the ICMJE uniform disclosure form (available at: http://dx.doi.org/10.21037/pcm-20-76). The series “Management of Triple Negative Breast Cancer” was commissioned by the editorial office without any funding or sponsorship. RF has following disclosures: Advisory Board or Honoraria: Merck, Novartis, Janssen, Pfizer, BMS, Bayer; Travel Grant: Janssen. AA has following disclosures: Advisory board: Eli Lily, Exact Sciences, Exactis, Novartis, Pfizer; Honoraria: Apotex, Roche; Travel: Roche. The authors have no other conflicts of interest to declare.
Ethical Statement: The authors are accountable for all aspects for the work in ensuring that questions related to the accuracy or integrity of any part of the work are appropriately investigated and resolved.
Open Access Statement: This is an Open Access article distributed in accordance with the Creative Commons Attribution-NonCommercial-NoDerivs 4.0 International License (CC BY-NC-ND 4.0), which permits the non-commercial replication and distribution of the article with the strict proviso that no changes or edits are made and the original work is properly cited (including links to both the formal publication through the relevant DOI and the license). See: https://creativecommons.org/licenses/by-nc-nd/4.0/.
References
- Carey LA, Perou CM, Livasy CA, et al. Race, breast cancer subtypes, and survival in the Carolina Breast Cancer Study. JAMA 2006;295:2492-502. [Crossref] [PubMed]
- O'Brien KM, Cole SR, Tse CK, et al. Intrinsic breast tumor subtypes, race, and long-term survival in the Carolina Breast Cancer Study. Clin Cancer Res 2010;16:6100-10. [Crossref] [PubMed]
- Foulkes WD, Stefansson IM, Chappuis PO, et al. Germline BRCA1 mutations and a basal epithelial phenotype in breast cancer. J Natl Cancer Inst 2003;95:1482-5. [Crossref] [PubMed]
- Dent R, Trudeau M, Pritchard KI, et al. Triple-negative breast cancer: clinical features and patterns of recurrence. Clin Cancer Res 2007;13:4429-34. [Crossref] [PubMed]
- Perou CM, Sørlie T, Eisen MB, et al. Molecular portraits of human breast tumours. Nature 2000;406:747-52. [Crossref] [PubMed]
- Prat A, Cruz C, Hoadley KA, et al. Molecular features of the basal-like breast cancer subtype based on BRCA1 mutation status. Breast Cancer Res Treat 2014;147:185-91. [Crossref] [PubMed]
- Cancer Genome Atlas Network. Comprehensive molecular portraits of human breast tumours. Nature 2012;490:61-70. [Crossref] [PubMed]
- Shah SP, Roth A, Goya R, et al. The clonal and mutational evolution spectrum of primary triple-negative breast cancers. Nature 2012;486:395-9. [Crossref] [PubMed]
- Schmid P, Rugo HS, Adams S, et al. Atezolizumab plus nab-paclitaxel as first-line treatment for unresectable, locally advanced or metastatic triple-negative breast cancer (IMpassion130): updated efficacy results from a randomised, double-blind, placebo-controlled, phase 3 trial. Lancet Oncol 2020;21:44-59. [Crossref] [PubMed]
- Cortes J, Cescon DW, Rugo HS, et al. Pembrolizumab plus chemotherapy versus placebo plus chemotherapy for previously untreated locally recurrent inoperable or metastatic triple-negative breast cancer (KEYNOTE-355): a randomised, placebo-controlled, double-blind, phase 3 clinical trial. Lancet 2020;396:1817-28. [Crossref] [PubMed]
- Litton JK, Rugo HS, Ettl J, et al. Talazoparib in Patients with Advanced Breast Cancer and a Germline BRCA Mutation. N Engl J Med 2018;379:753-63. [Crossref] [PubMed]
- Robson M, Goessl C, Domchek S. Olaparib for Metastatic Germline BRCA-Mutated Breast Cancer. N Engl J Med 2017;377:1792-3. [Crossref] [PubMed]
- Robson ME, Tung N, Conte P, et al. OlympiAD final overall survival and tolerability results: Olaparib versus chemotherapy treatment of physician's choice in patients with a germline BRCA mutation and HER2-negative metastatic breast cancer. Ann Oncol 2019;30:558-66. [Crossref] [PubMed]
- Bardia A, Mayer IA, Vahdat LT, et al. Sacituzumab Govitecan-hziy in Refractory Metastatic Triple-Negative Breast Cancer. N Engl J Med. 2019;380:741-51. [Crossref] [PubMed]
- News DR. ASCENT: A Landmark Advance in Treating Refractory Triple-Negative Breast Cancer [Dec 14, 2020]. Available online: https://www.esmo.org/meetings/past-meetings/esmo-virtual-congress-2020/daily-reporter/daily-reporter-news/ascent-a-landmark-advance-in-treating-refractory-triple-negative-breast-cancer
- Lim E, Vaillant F, Wu D, et al. Aberrant luminal progenitors as the candidate target population for basal tumor development in BRCA1 mutation carriers. Nat Med 2009;15:907-13. [Crossref] [PubMed]
- Molyneux G, Geyer FC, Magnay FA, et al. BRCA1 basal-like breast cancers originate from luminal epithelial progenitors and not from basal stem cells. Cell Stem Cell 2010;7:403-17. [Crossref] [PubMed]
- Visvader JE, Stingl J. Mammary stem cells and the differentiation hierarchy: current status and perspectives. Genes Dev 2014;28:1143-58. [Crossref] [PubMed]
- Sørlie T, Perou CM, Tibshirani R, et al. Gene expression patterns of breast carcinomas distinguish tumor subclasses with clinical implications. Proc Natl Acad Sci U S A 2001;98:10869-74. [Crossref] [PubMed]
- Prat A, Fan C, Fernandez A, et al. Response and survival of breast cancer intrinsic subtypes following multi-agent neoadjuvant chemotherapy. BMC Med 2015;13:303. [Crossref] [PubMed]
- Prat A, Parker JS, Karginova O, et al. Phenotypic and molecular characterization of the claudin-low intrinsic subtype of breast cancer. Breast Cancer Res 2010;12:R68. [Crossref] [PubMed]
- Prat A, Perou CM. Deconstructing the molecular portraits of breast cancer. Mol Oncol 2011;5:5-23. [Crossref] [PubMed]
- Sorlie T, Tibshirani R, Parker J, et al. Repeated observation of breast tumor subtypes in independent gene expression data sets. Proc Natl Acad Sci U S A 2003;100:8418-23. [Crossref] [PubMed]
- Lehmann BD, Bauer JA, Chen X, et al. Identification of human triple-negative breast cancer subtypes and preclinical models for selection of targeted therapies. J Clin Invest 2011;121:2750-67. [Crossref] [PubMed]
- Lehmann BD, Jovanović B, Chen X, et al. Refinement of Triple-Negative Breast Cancer Molecular Subtypes: Implications for Neoadjuvant Chemotherapy Selection. PLoS One 2016;11:e0157368. [Crossref] [PubMed]
- Jiang YZ, Ma D, Suo C, et al. Genomic and Transcriptomic Landscape of Triple-Negative Breast Cancers: Subtypes and Treatment Strategies. Cancer Cell 2019;35:428-40.e5. [Crossref] [PubMed]
- Lehmann BD, Pietenpol JA. Identification and use of biomarkers in treatment strategies for triple-negative breast cancer subtypes. J Pathol 2014;232:142-50. [Crossref] [PubMed]
- Masuda H, Baggerly KA, Wang Y, et al. Differential response to neoadjuvant chemotherapy among 7 triple-negative breast cancer molecular subtypes. Clin Cancer Res 2013;19:5533-40. [Crossref] [PubMed]
- Yu KD, Zhu R, Zhan M, et al. Identification of prognosis-relevant subgroups in patients with chemoresistant triple-negative breast cancer. Clin Cancer Res 2013;19:2723-33. [Crossref] [PubMed]
- Echavarria I, López-Tarruella S, Picornell A, et al. Pathological Response in a Triple-Negative Breast Cancer Cohort Treated with Neoadjuvant Carboplatin and Docetaxel According to Lehmann's Refined Classification. Clin Cancer Res 2018;24:1845-52. [Crossref] [PubMed]
- Burstein MD, Tsimelzon A, Poage GM, et al. Comprehensive genomic analysis identifies novel subtypes and targets of triple-negative breast cancer. Clin Cancer Res 2015;21:1688-98. [Crossref] [PubMed]
- Garrido-Castro AC, Lin NU, Polyak K. Insights into Molecular Classifications of Triple-Negative Breast Cancer: Improving Patient Selection for Treatment. Cancer Discov 2019;9:176-98. [Crossref] [PubMed]
- Gao J, Aksoy BA, Dogrusoz U, et al. Integrative analysis of complex cancer genomics and clinical profiles using the cBioPortal. Sci Signal 2013;6:pl1. [Crossref] [PubMed]
- Muller PA, Vousden KH. Mutant p53 in cancer: new functions and therapeutic opportunities. Cancer Cell 2014;25:304-17. [Crossref] [PubMed]
- Liu X, Holstege H, van der Gulden H, et al. Somatic loss of BRCA1 and p53 in mice induces mammary tumors with features of human BRCA1-mutated basal-like breast cancer. Proc Natl Acad Sci U S A 2007;104:12111-6. [Crossref] [PubMed]
- Tao L, Xiang D, Xie Y, et al. Induced p53 loss in mouse luminal cells causes clonal expansion and development of mammary tumours. Nat Commun. 2017;8:14431. [Crossref] [PubMed]
- Abba MC, Gong T, Lu Y, et al. A Molecular Portrait of High-Grade Ductal Carcinoma In Situ. Cancer Res 2015;75:3980-90. [Crossref] [PubMed]
- Chae BJ, Bae JS, Lee A, et al. p53 as a specific prognostic factor in triple-negative breast cancer. Jpn J Clin Oncol 2009;39:217-24. [Crossref] [PubMed]
- Darb-Esfahani S, Denkert C, Stenzinger A, et al. Role of TP53 mutations in triple negative and HER2-positive breast cancer treated with neoadjuvant anthracycline/taxane-based chemotherapy. Oncotarget 2016;7:67686-98. [Crossref] [PubMed]
- Li JP, Zhang XM, Zhang Z, et al. Association of p53 expression with poor prognosis in patients with triple-negative breast invasive ductal carcinoma. Medicine (Baltimore) 2019;98:e15449. [Crossref] [PubMed]
- Pan Y, Yuan Y, Liu G, et al. P53 and Ki-67 as prognostic markers in triple-negative breast cancer patients. PLoS One 2017;12:e0172324. [Crossref] [PubMed]
- Bae SY, Nam SJ, Jung Y, et al. Differences in prognosis and efficacy of chemotherapy by p53 expression in triple-negative breast cancer. Breast Cancer Res Treat 2018;172:437-44. [Crossref] [PubMed]
- Synnott NC, Murray A, McGowan PM, et al. Mutant p53: a novel target for the treatment of patients with triple-negative breast cancer? Int J Cancer 2017;140:234-46. [Crossref] [PubMed]
- Synnott NC, O'Connell D, Crown J, et al. COTI-2 reactivates mutant p53 and inhibits growth of triple-negative breast cancer cells. Breast Cancer Res Treat 2020;179:47-56. [Crossref] [PubMed]
- Kensler KH, Regan MM, Heng YJ, et al. Prognostic and predictive value of androgen receptor expression in postmenopausal women with estrogen receptor-positive breast cancer: results from the Breast International Group Trial 1-98. Breast Cancer Res 2019;21:30. [Crossref] [PubMed]
- Kensler KH, Poole EM, Heng YJ, et al. Androgen Receptor Expression and Breast Cancer Survival: Results From the Nurses' Health Studies. J Natl Cancer Inst 2019;111:700-8. [Crossref] [PubMed]
- Xu M, Yuan Y, Yan P, et al. Prognostic Significance of Androgen Receptor Expression in Triple Negative Breast Cancer: A Systematic Review and Meta-Analysis. Clin Breast Cancer 2020;20:e385-96. [Crossref] [PubMed]
- Gucalp A, Tolaney S, Isakoff SJ, et al. Phase II trial of bicalutamide in patients with androgen receptor-positive, estrogen receptor-negative metastatic Breast Cancer. Clin Cancer Res 2013;19:5505-12. [Crossref] [PubMed]
- Bonnefoi H, Grellety T, Tredan O, et al. A phase II trial of abiraterone acetate plus prednisone in patients with triple-negative androgen receptor positive locally advanced or metastatic breast cancer (UCBG 12-1). Ann Oncol 2016;27:812-8. [Crossref] [PubMed]
- Traina TA, Miller K, Yardley DA, et al. Enzalutamide for the Treatment of Androgen Receptor-Expressing Triple-Negative Breast Cancer. J Clin Oncol 2018;36:884-90. [Crossref] [PubMed]
- Asghar US, Barr AR, Cutts R, et al. Single-Cell Dynamics Determines Response to CDK4/6 Inhibition in Triple-Negative Breast Cancer. Clin Cancer Res 2017;23:5561-72. [Crossref] [PubMed]
- Moynahan ME, Chiu JW, Koller BH, et al. Brca1 controls homology-directed DNA repair. Mol Cell 1999;4:511-8. [Crossref] [PubMed]
- Antoniou A, Pharoah PD, Narod S, et al. Average risks of breast and ovarian cancer associated with BRCA1 or BRCA2 mutations detected in case Series unselected for family history: a combined analysis of 22 studies. Am J Hum Genet 2003;72:1117-30. [Crossref] [PubMed]
- Chen S, Parmigiani G. Meta-analysis of BRCA1 and BRCA2 penetrance. J Clin Oncol 2007;25:1329-33. [Crossref] [PubMed]
- Narod SA, Foulkes WD. BRCA1 and BRCA2: 1994 and beyond. Nat Rev Cancer 2004;4:665-76. [Crossref] [PubMed]
- Kuchenbaecker KB, Hopper JL, Barnes DR, et al. Risks of Breast, Ovarian, and Contralateral Breast Cancer for BRCA1 and BRCA2 Mutation Carriers. JAMA 2017;317:2402-16. [Crossref] [PubMed]
- Mavaddat N, Barrowdale D, Andrulis IL, et al. Pathology of breast and ovarian cancers among BRCA1 and BRCA2 mutation carriers: results from the Consortium of Investigators of Modifiers of BRCA1/2 (CIMBA). Cancer Epidemiol Biomarkers Prev 2012;21:134-47. [Crossref] [PubMed]
- Krammer J, Pinker-Domenig K, Robson ME, et al. Breast cancer detection and tumor characteristics in BRCA1 and BRCA2 mutation carriers. Breast Cancer Res Treat 2017;163:565-71. [Crossref] [PubMed]
- Foulkes WD, Metcalfe K, Sun P, et al. Estrogen receptor status in BRCA1- and BRCA2-related breast cancer: the influence of age, grade, and histological type. Clin Cancer Res 2004;10:2029-34. [Crossref] [PubMed]
- Tutt A, Tovey H, Cheang MCU, et al. Carboplatin in BRCA1/2-mutated and triple-negative breast cancer BRCAness subgroups: the TNT Trial. Nat Med 2018;24:628-37. [Crossref] [PubMed]
- Drost R, Bouwman P, Rottenberg S, et al. BRCA1 RING function is essential for tumor suppression but dispensable for therapy resistance. Cancer Cell 2011;20:797-809. [Crossref] [PubMed]
- Edwards SL, Brough R, Lord CJ, et al. Resistance to therapy caused by intragenic deletion in BRCA2. Nature 2008;451:1111-5. [Crossref] [PubMed]
- Atipairin A, Ratanaphan A. In Vitro Enhanced Sensitivity to Cisplatin in D67Y BRCA1 RING Domain Protein. Breast Cancer (Auckl) 2011;5:201-8. [Crossref] [PubMed]
- Ashworth A, Lord CJ. Synthetic lethal therapies for cancer: what's next after PARP inhibitors? Nat Rev Clin Oncol 2018;15:564-76. [Crossref] [PubMed]
- Gudmundsdottir K, Ashworth A. The roles of BRCA1 and BRCA2 and associated proteins in the maintenance of genomic stability. Oncogene 2006;25:5864-74. [Crossref] [PubMed]
- Lord CJ, Ashworth A. PARP inhibitors: Synthetic lethality in the clinic. Science 2017;355:1152-8. [Crossref] [PubMed]
- Diéras V, Han HS, Kaufman B, et al. Veliparib with carboplatin and paclitaxel in BRCA-mutated advanced breast cancer (BROCADE3): a randomised, double-blind, placebo-controlled, phase 3 trial. Lancet Oncol 2020;21:1269-82. [Crossref] [PubMed]
- Mouw KW, Goldberg MS, Konstantinopoulos PA, et al. DNA Damage and Repair Biomarkers of Immunotherapy Response. Cancer Discov 2017;7:675-93. [Crossref] [PubMed]
- Davies H, Glodzik D, Morganella S, et al. HRDetect is a predictor of BRCA1 and BRCA2 deficiency based on mutational signatures. Nat Med 2017;23:517-25. [Crossref] [PubMed]
- Glodzik D, Bosch A, Hartman J, et al. Comprehensive molecular comparison of BRCA1 hypermethylated and BRCA1 mutated triple negative breast cancers. Nat Commun 2020;11:3747. [Crossref] [PubMed]
- Lin PH, Chen M, Tsai LW, et al. Using next-generation sequencing to redefine BRCAness in triple-negative breast cancer. Cancer Sci 2020;111:1375-84. [Crossref] [PubMed]
- Watkins JA, Irshad S, Grigoriadis A, et al. Genomic scars as biomarkers of homologous recombination deficiency and drug response in breast and ovarian cancers. Breast Cancer Res 2014;16:211. [Crossref] [PubMed]
- Polak P, Kim J, Braunstein LZ, et al. A mutational signature reveals alterations underlying deficient homologous recombination repair in breast cancer. Nat Genet 2017;49:1476-86. [Crossref] [PubMed]
- Telli ML, Timms KM, Reid J, et al. Homologous Recombination Deficiency (HRD) Score Predicts Response to Platinum-Containing Neoadjuvant Chemotherapy in Patients with Triple-Negative Breast Cancer. Clin Cancer Res 2016;22:3764-73. [Crossref] [PubMed]
- Tung NM, Robson ME, Ventz S, et al. TBCRC 048: Phase II Study of Olaparib for Metastatic Breast Cancer and Mutations in Homologous Recombination-Related Genes. J Clin Oncol. 2020;38:JCO2002151. [Crossref] [PubMed]
- Cantley LC. The phosphoinositide 3-kinase pathway. Science 2002;296:1655-7. [Crossref] [PubMed]
- Fedele CG, Ooms LM, Ho M, et al. Inositol polyphosphate 4-phosphatase II regulates PI3K/Akt signaling and is lost in human basal-like breast cancers. Proc Natl Acad Sci U S A 2010;107:22231-6. [Crossref] [PubMed]
- Liu H, Paddock MN, Wang H, et al. The INPP4B Tumor Suppressor Modulates EGFR Trafficking and Promotes Triple-Negative Breast Cancer. Cancer Discov 2020;10:1226-39. [Crossref] [PubMed]
- Beg S, Siraj AK, Prabhakaran S, et al. Loss of PTEN expression is associated with aggressive behavior and poor prognosis in Middle Eastern triple-negative breast cancer. Breast Cancer Res Treat 2015;151:541-53. [Crossref] [PubMed]
- Mosele F, Stefanovska B, Lusque A, et al. Outcome and molecular landscape of patients with PIK3CA-mutated metastatic breast cancer. Ann Oncol 2020;31:377-86. [Crossref] [PubMed]
- Kim SB, Dent R, Im SA, et al. Ipatasertib plus paclitaxel versus placebo plus paclitaxel as first-line therapy for metastatic triple-negative breast cancer (LOTUS): a multicentre, randomised, double-blind, placebo-controlled, phase 2 trial. Lancet Oncol 2017;18:1360-72. [Crossref] [PubMed]
- Bennett C. Addition of Ipatasertib Confers No Benefit in Advanced Triple-Negative Breast Cancer 2020 [Dec 14 2020]. Available online: https://www.cancertherapyadvisor.com/home/news/conference-coverage/san-antonio-breast-cancer-symposium-sabcs/sabcs-2020/breast-cancer-tnbc-ipatasertib-no-benefit-treatment-risk/
- Schmid P, Loirat D, Savas P, et al. Abstract CT049: Phase Ib study evaluating a triplet combination of ipatasertib (IPAT), atezolizumab (atezo), and paclitaxel (PAC) or nab-PAC as first-line (1L) therapy for locally advanced/metastatic triple-negative breast cancer (TNBC), July 2019. Available online: https://cancerres.aacrjournals.org/content/79/13_Supplement/CT049
- Schmid P, Abraham J, Chan S, et al. Capivasertib Plus Paclitaxel Versus Placebo Plus Paclitaxel As First-Line Therapy for Metastatic Triple-Negative Breast Cancer: The PAKT Trial. J Clin Oncol 2020;38:423-33. [Crossref] [PubMed]
- Sivapiragasam A, Ashok Kumar P, Sokol ES, et al. Predictive Biomarkers for Immune Checkpoint Inhibitors in Metastatic Breast Cancer. Cancer Med 2021;10:53-61. [Crossref] [PubMed]
- Ghahremanloo A, Soltani A, Modaresi SMS, et al. Recent advances in the clinical development of immune checkpoint blockade therapy. Cell Oncol (Dordr) 2019;42:609-26. [Crossref] [PubMed]
- Schmid P, Adams S, Rugo HS, et al. Atezolizumab and Nab-Paclitaxel in Advanced Triple-Negative Breast Cancer. N Engl J Med 2018;379:2108-21. [Crossref] [PubMed]
- Li Y, Vennapusa B, Chang CW, et al. Prevalence Study of PD-L1 SP142 Assay in Metastatic Triple-negative Breast Cancer. Appl Immunohistochem Mol Morphol 2021;29:258-64. [Crossref] [PubMed]
- Zhang M, Sun H, Zhao S, et al. Expression of PD-L1 and prognosis in breast cancer: a meta-analysis. Oncotarget 2017;8:31347-54. [Crossref] [PubMed]
- Wang X, Liu Y. PD-L1 expression in tumor infiltrated lymphocytes predicts survival in triple-negative breast cancer. Pathol Res Pract 2020;216:152802. [Crossref] [PubMed]
- Nanda R, Chow LQ, Dees EC, et al. Pembrolizumab in Patients With Advanced Triple-Negative Breast Cancer: Phase Ib KEYNOTE-012 Study. J Clin Oncol 2016;34:2460-7. [Crossref] [PubMed]
- Adams S, Schmid P, Rugo HS, et al. Pembrolizumab monotherapy for previously treated metastatic triple-negative breast cancer: cohort A of the phase II KEYNOTE-086 study. Ann Oncol 2019;30:397-404. [Crossref] [PubMed]
- Adams S, Loi S, Toppmeyer D, et al. Pembrolizumab monotherapy for previously untreated, PD-L1-positive, metastatic triple-negative breast cancer: cohort B of the phase II KEYNOTE-086 study. Ann Oncol 2019;30:405-11. [Crossref] [PubMed]
- Cortés J, Lipatov O, Im SA, et al. LBA21 - KEYNOTE-119: Phase III study of pembrolizumab (pembro) versus single-agent chemotherapy (chemo) for metastatic triple negative breast cancer (mTNBC). Ann Oncol 2019;30:v859-60. [Crossref]
- Adams S, Diamond JR, Hamilton E, et al. Atezolizumab Plus nab-Paclitaxel in the Treatment of Metastatic Triple-Negative Breast Cancer With 2-Year Survival Follow-up: A Phase 1b Clinical Trial. JAMA Oncol 2019;5:334-42. [Crossref] [PubMed]
- Helwick C. IMpassion131: No Benefit for Atezolizumab Plus Paclitaxel in Triple-Negative Breast Cancer 2020. Available online: https://ascopost.com/issues/october-10-2020/impassion131-no-benefit-for-atezolizumab-plus-paclitaxel-in-triple-negative-breast-cancer/
- Lotfinejad P, Asghari Jafarabadi M, Abdoli Shadbad M, et al. Prognostic Role and Clinical Significance of Tumor-Infiltrating Lymphocyte (TIL) and Programmed Death Ligand 1 (PD-L1) Expression in Triple-Negative Breast Cancer (TNBC): A Systematic Review and Meta-Analysis Study. Diagnostics (Basel) 2020;10:704. [Crossref] [PubMed]
- Lee JS, Ruppin E. Multiomics Prediction of Response Rates to Therapies to Inhibit Programmed Cell Death 1 and Programmed Cell Death 1 Ligand 1. JAMA Oncol 2019;5:1614-8. [Crossref] [PubMed]
- Taylor KM, Morgan HE, Johnson A, et al. Structure-function analysis of LIV-1, the breast cancer-associated protein that belongs to a new subfamily of zinc transporters. Biochem J 2003;375:51-9. [Crossref] [PubMed]
- Modi S, Pusztai L, Forero A, et al. Phase 1 study of the antibody–drug conjugate SGN-LIV1A in patients with heavily pretreated triple-negative metastatic breast cancer. 2018 [Dec 14 2020]. Available online: https://www.researchgate.net/publication/323212556_Abstract_PD3-14_Phase_1_study_of_the_antibody-drug_conjugate_SGN-LIV1A_in_patients_with_heavily_pretreated_triple-negative_metastatic_breast_cancer
- Modi S, Park H, Murthy RK, et al. Antitumor Activity and Safety of Trastuzumab Deruxtecan in Patients With HER2-Low-Expressing Advanced Breast Cancer: Results From a Phase Ib Study. J Clin Oncol 2020;38:1887-96. [Crossref] [PubMed]
Cite this article as: Chehade R, Awan AA, Fernandes R. A narrative review of biomarkers in advanced triple negative breast cancer. Precis Cancer Med 2021;4:24.