ROS-1 NSCLC therapy resistance mechanism
Introduction
ROS1 function
ROS proto-oncogene 1, receptor tyrosine kinase gene (c-ROS-1 or ROS1) was originally identified in 1982 as the oncogenic part of the avian sarcoma RNA virus UR2 (1-3). ROS1 gene is located on chromosome 6q22.1 (4,5); the unaltered gene encodes a transmembrane protein with intracellular C-terminal tyrosine kinase domain (6) that shares marked sequence homology and structural similarities to anaplastic lymphoma kinase (ALK), leukocyte tyrosine kinase (LTK), and the insulin receptor families (7). In animals, ROS1 expression has been detected in the epithelial cells of several organs. Interestingly, a Ros1-deficient male mouse was found to be sterile, due to defective differentiation of epididymal epithelial cells; while, female Ros1-deficient mice developed no anomalies (8-10). Recently, Kiyozumi et al. observed that the extracellular domain of the mouse ROS1 receptor attaches to neural epidermal growth factor-like like 2 (NELL2), a testicular germ cell-secreted lumicrine factor (11).
In humans, ROS1 is highly expressed in the lungs. Functional activation of human ROS1 receptor via NELL2 is suspected given the results of the aforementioned studies but has not been proven. Therefore, the role of native ROS1 in humans has not been yet well established (10).
ROS1 gene fusions in cancer
ROS1 gene is sensitive to chromosomal rearrangements ending in fusion proteins that involve the expression of active ROS1 tyrosine kinase domain. ROS1 rearrangements were initially identified in a human glioblastoma cell line (12) and are involved in the pathogenesis of other malignancies including cholangiocarcinoma, ovarian cancer, gastric cancer, colorectal cancer, angiosarcoma, inflammatory myofibroblastic tumor, Spitz tumors, epithelioid haemangioendothelioma and lung cancer (13-21).
The precise mechanism by which ROS1 fusion proteins become constitutively active is unclear. Indeed, many of the common ROS1 fusion partners do not have dimerization domains. Moreover, subcellular localization varies upon fusion partner and has been described not exclusively as a transmembrane protein but also in the cytoplasmic compartment only as well as in the reticular endoplasmic membrane. Once activated, ROS1 kinase activates the SHP-2 phosphatase and upregulates MAPK/ERK, PI3K/AKT/mTOR and JAK/STAT3 signaling pathways to promote cell growth and survival (6,8,16,22-24).
ROS1-rearranged NSCLC
Chromosomal rearrangements involving ROS1 gene were initially described in non-small cell lung cancer (NSCLC) in 2007 by Rikova et al. (25). Such rearrangements arise in 1–2% of advanced NSCLC and define a distinct molecular subgroup in NSCLC (8,26,27). Most of break points in ROS1 occur in a variable region among exons 32 and 35, conserving the kinase domain encoded in 3’ (27,28). So far, over 14 different fusion partner genes have been reported in NSCLC patients, including CD74, SLC34A2, SDC4, EZR, FIG, TPM3, LRIG3, KDELR2, CCDC6, MSN, TMEM106B, TPD52L, CLTC, and LIMA1. Of these fusion partners, CD74-ROS1 is the most common variant in ROS1-rearranged NSCLC (~44%), followed by EZR–ROS1 (16%), SDC4–ROS1 (14%), SLC34A2–ROS1 (10%), and TPM3-ROS1 (8%) (8,10). Contemporary studies suggest that the type of the ROS1 fusion partner may influence the prognosis and treatment response in patients with advanced ROS1-rearranged NSCLC. In fact, patients with CD74-ROS1 rearrangements had a poorer prognosis than those without CD74-ROS1 fusions (29,30).
Similar to patients with NSCLC harboring ALK fusions, patients with ROS1-rearranged NSCLC are usually younger, light or never smokers, and the predominant histology is adenocarcinoma (8,26). However, compared to patients with ALK-rearranged NSCLC, ROS1-rearranged NSCLC patients tend to develop lower rates of extrathoracic and brain metastases at initial diagnosis, and have a lower cumulative incidence of brain metastases (31). Additionally, the incidence of venous thromboembolism (VTE) seems to be higher in patients with NSCLC harboring ROS1-fusions than previously observed for the general population with NSCLC (32,33).
ROS1 fusions rarely co-exist with other driver mutations, such as KRAS, EGFR or ALK (30,34). ROS1 fusions have also been described as a mechanism of resistance to targeted therapies in EGFR mutant lung cancer patients, being this a rare event (35).
Diagnosing ROS1 rearrangements
Most guidelines recommended ROS1 testing for all advanced-stage lung adenocarcinomas, regardless of clinical characteristics (36-38).
ROS1 fusions can be detected in tumor tissue by different techniques including immunohistochemistry (IHC), reverse transcriptase polymerase chain reaction (PCR), fluorescence in-situ hybridization (FISH), and next generation sequencing (NGS).
FISH
ROS1 FISH has been the standard method for detecting ROS1 gene fusions, as it was the diagnosis method used in initial clinical trials with crizotinib.
Using fluorescently labelled, paired break-apart probes is considered the “gold standard” in detecting ROS1 rearrangement. Positivity is defined as >15% of tumor cells containing the classic split 3' and 5', or the atypical isolated 3' signals (8,39).
FISH can be performed on a small amount of tissue, and without previous knowledge of fusion partners. However, it is expensive, technically challenging and hard to interpret, and both false-positive (non-functional fusions or isolated 3´signals) and false negative results (complex staining patterns or intra-chromosal microdeletions) can occur (39).
IHC
Immunohistochemistry can be used to diagnose ROS1 fusions, and it is considered a cheaper and faster technique compared with FISH.
However, the ROS1 IHC results can be difficult to interpret. ROS1 staining patterns may vary and IHC may be falsely negative or falsely positive due to several reasons (different intracellular localization of the ROS1 fusions, ROS1 expression from benign pneumocytes and alveolar macrophages, or aneuploidy leading to aberrant expression) (8). Therefore, ROS1 IHC requires verification using a second techniques, such as NGS or FISH.
Reverse transcriptase PCR (RT-PCR)
Combined with NGS or Sanger, RT-PCR allows detection of ROS1 fusion partners. RNA sequencing needs to be converted to complementary DNA by reverse transcription and PCR amplification, yet potential errors can be easily introduced when manipulating short or very fragmented DNA material. Additionally, RT-PCR requires a previous knowledge of fusions for developing fusion-specific primers; therefore, unknown fusions can be missed, which limits its use (8).
Next generation sequencing (NGS)
Next-generation sequencing allows either DNA, RNA or both DNA and RNA-based nucleic acid sequencing of multiple genes simultaneously to identify any ROS1 fusion, in addition to other driver mutations.
Tumor DNA enrichment can be completed via amplicon-based or hybrid capture-based approaches. Hybrid capture can sequence wider regions of the genome and better detect the fusion partner gene; therefore, is the preferred method to detect ROS1 fusion (10).
NGS can be done on tumor tissue-derived DNA or plasma circulating tumor DNA (ctDNA). Liquid biopsy can detect ROS1 fusions, but this approach can be challenging because of variations of DNA shedding rates that drive the concentration of ctDNA in the blood (10).
However, DNA-based NGS can fail to identify an oncogenic driver due to the lack of capacity of cover intronic breakpoints containing numerous repetitive elements.
RNA-based NGS can overcome this limitation of DNA-based NGS by sequencing coding regions instead of introns (40). Moreover, RNA-sequencing enables detection of any fused partner and the discovery of new ones. These advantages of RNA NGS are limited by the need of high RNA quality, that could be low in clinical samples, particularly in FFPE samples.
Additionally, there are some limitations of NGS. It is slower and more expensive than IHC or FISH, and tissue requirements are higher. Nevertheless, because of its capacity to detect both known and unknown fusions, and sequence multiple genes at the same time, NGS is increasingly being used and will likely become the standard tool for ROS1 fusion detection in the future (39).
Molecules targeting ROS1 in NSCLC: crizotinib
The identification of oncogenic driver mutations in NSCLC involved the development of molecularly targeted therapy, changing the standard of care for patients harboring these driver mutations (8,41). Tyrosine kinase inhibitors (TKIs) with activity against ROS1 fusion proteins have allowed for dramatic improvements in objective response rate (ORR), progression-free survival (PFS), overall survival (OS) and quality of life compared with chemotherapy (42,43).
Crizotinib
Crizotinib is a multi-targeted kinase inhibitor with affinity for ALK, ROS and MET (44).
There is structural equivalence between the ALK and ROS1 kinase domains, resulting in cross-inhibition between current therapies targeted against these kinases (45,46).
Crizotinib is a strong ROS1 inhibitor (44) which blocks ATP dependent cellular processes, developing a complex with the respective protein kinase domains, and inhibiting of ROS1 phosphorylation, leading to cell apoptosis with dose-dependent efficacy showed in cells with SLC34A2-ROS1 translocations (39,44).
In a phase I study (PROFILE 1001; NCT00585195 (47) enrolling 50 patients with ROS1-rearranged advanced NSCLC treated with crizotinib, ORR was 70%. Median progression-free survival (PFS) was 19.2 months and the disease control rate (DCS) was 90%. Responses to crizotinib were detected regardless of the ROS1 fusion partner. Median OS was reported to be 51.4 months, one of the most impressive median overall survival found in any prospective trial of targeted therapy for advanced NSCLCs. The safety profile of crizotinib was favorable, similar to the observed for patients with ALK positive NSCLC.
After the efficacy and safety results from PROFILE-1001 study, crizotinib became the first targeted therapy approved by both the U.S. Food and Drug Administration (FDA) and the European Medicines Agency (EMA) in 2016 for the treatment of advanced ROS1-rearranged NSCLC. Moreover, in 2017, both the National Comprehensive Cancer Network (NCCN) and European Society of Medical Oncology (ESMO) guidelines recommend crizotinib treatment for patients with advanced/metastatic lung cancer with known ROS1 rearrangements (38,48).
Other studies subsequently confirmed the efficacy of crizotinib in ROS1-rearranged NSCLC, including two phase II studies in European and East Asian population (49-51). Interestingly, the median PFS duration of patients receiving crizotinib is longer for patients harboring ROS1 NSCLC than for patients with ALK fusions, possibly owing to more-potent inhibition of ROS1 with crizotinib compared with ALK (31). However, most patients treated with crizotinib invariably relapse. The management of crizotinib-resistant ROS1-rearranged NSCLC represents a significant challenge.
Resistance mechanisms to crizotinib
Mechanisms of crizotinib resistance share features with other TKIs and include pharmacokinetic/dynamic failure, biological acquired resistance by secondary point mutations in the ROS1 kinase domain, bypass tracks, and phenotypic changes (Figure 1) (52-54).
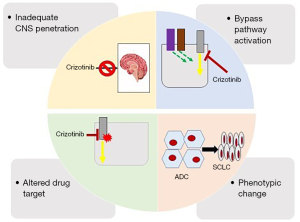
Intracranial failure
Crizotinib has limited blood–brain barrier (BBB) penetration as it is a substrate of P-glycoprotein and human ATP-binding cassette subfamily efflux transporters (55). Therefore, most patients treated with crizotinib frequently relapse with new brain progression or progression of existing intracranial disease (39). CNS progression to crizotinib might reflect a pharmacokinetic failure of therapy rather than true biological resistance. Cerebrospinal fluid (CSF) concentrations of crizotinib are usually low, and the intracranial ORR with this agent was only 33% (10,55,56).
The estimated incidence of brain metastases in advanced NSCLC is between 20% and 40% prior to start treatment, and can increase to up to 30−50% in patients pre-treated with TKIs in ROS1+ lung cancer patients (57).
Novel ROS1 inhibitors have been designed to better penetrate the BBB and achieve a higher drug CNS concentration, obtaining higher efficacy for patients with brain metastasis. In metastatic patients in first-line setting, entrectinib (intracranial ORR 55%), lorlatinib (iORR 64%) and repotrectinib (iORR 100%) showed substantial intracranial activity in patients with treatment naïve ROS1-rearranged NSCLC (58-60). Entrectinib is now approved by both the FDA and EMA in ROS-1 rearranged NSCLC in first line and is a treatment to consider in patients with brain metastases at diagnosis.
Notably, the FDA has granted repotrectinib fast-track designation for the treatment of ROS1-rearranged NSCLC patients previously treated with one prior ROS1 TKI and a platinum-doublet, being a potential second-line treatment option after CNS or systemic progression to crizotinib.
On-target resistance (ROS1-intrinsic mechanisms) (Table 1)
Table 1
Mutation | Protein location | Proposed mechanism of resistance | ROS TKI with activity against resistance mutation |
---|---|---|---|
G2032R | Solvent front of the kinase hinge | Glycine-to-arginine substitution at codon 2031 in the ROS1 kinase domain causes resistance to RO1 kinase inhibition through steric interference with drug binding | Cabozantinib (61) |
Repotrectinib (62) | |||
Taletrectinib (63)* | |||
Lorlatinib (64)* | |||
D2033N | Solvent front of the kinase hinge | Aspartic acid-to-asparagine substitution at codon 2033 leads to a modification of electrostatic forces at the exterior surface of the ATP-binding site and reorientation of surrounding residues | Lorlatinib (65)* |
Cabozantinib (66) | |||
Repotrectinib (62) | |||
L2026M | Gatekeeper position of inhibitor binding pocket | Leucine-to-methionine substitution at codon 2026 | Lorlatinib (59)* |
Ceritinib (24)* | |||
Brigatinib (24)* | |||
Cabozantinib (61,67)* | |||
Repotrectinib (68)* | |||
S1986F/Y | Not reported | Serine to tyrosine (S1986Y)/serine to phenylalaline (S1986F) substitution leads to an obstruction in the path to the enzyme active site and an increase in kinase activity | Lorlatinib (24)* |
*in vitro only.
Secondary point mutations within the ROS1 kinase domain have been identified in both clinical and preclinical studies, occurring approximately in 50−60% of crizotinib resistant tumors (31).
Several of these mutations are analogous to ALK secondary mutations that arise with ALK inhibitors, although secondary point mutations occur more frequently in crizotinib resistant ROS1 rearranged NSCLC, probably due to a higher inhibitory potency against ROS1 (10,39,47). These acquired mutations significantly reduce the potency of the inhibitor against the active ROS1 kinase by steric hindrance and it can also affect the sensitivity to other ROS1 TKIs. Thus, one strategy to overcome crizotinib-resistance could be the identification of the type of secondary mutation in order to select an effective targeted therapy in second line capable of inhibiting the mutated target (69).
G2032R
The first documented and most reported ROS1 secondary mutation observed in patients to date is the ROS1 G2032R mutation. It was the first crizotinib-resistant mechanism discovered in a patient with ROS1-rearranged NSCLC (70). Several small series had identified G2032R as one of the most frequent resistance mutations (31).
The G2032R mutation, a glycine-to-arginine substitution at codon 2032 in the solvent-front, causes resistance to crizotinib through steric interference with the drug binding at ROS1-kinase residues exposed to solvent (39,69,70). It is structurally similar to the ALK-G1202R mutation, making it difficult to inhibit pharmacologically (46,70).
Additionally, ROS1 G2032R can lead to epithelial-mesenchymal transition (EMT) and to enhance the invasive capacities of ROS1 fusion-driven cancer cells through upregulation of Twist1 (10,71).
D2033N
D2033N mutation, a substitution of an aspartic acid-to-asparagine at ROS1 2033 codon, is analogous to ALK D1203N (52,69). As the G2032R mutation previously described, D2033N mutation arises at the solvent-front region of the ATP-binding site of ROS1, affecting key electrostatic interaction between the D2033 residue and the piperidine moiety of crizotinib, and the surrounding residues at the surface of the ATP-binding pocket (39,66).
L2026M
L2026M involves leucine and methionine and it is analogous to ALK L1196M. It is located at the “gatekeeper” position of the ATP-binding pocket which hinders drug binding, conferring crizotinib resistance (53).
S1986Y/F
S1986Y/F mutations (substitution of the serine at 1986 ROS1 position by either tyrosine (S1986Y) or phenylalanine (S1986F) induces a positional change in the αC helix of the kinase domain which causes steric interference with drug binding. It is analogous to ALK C1156Y (52).
Other secondary mutations reported in crizotinib resistant ROS1-rearranged NSCLC include E1935G, L1947R, L1951R, G1971E, L1982F, C2060G, V2098I, and L2155 (10).
Off-target resistance (ROS1- extrinsic mechanisms)
By-pass tracks
Resistance to TKIs can develop via ROS1-extrinsic resistance mechanisms.
Activation of bypass-signaling have been reported for crizotinib resistance in ROS1 rearranged NSCLC (34). Tumor cells under the pressure of the targeted TKI can become resistant through upregulation of either downstream or parallel cell signaling pathways like EGFR, MET, HER2, KRAS, KIT, BRAF and MEK (10,31,52,53,66).
Retrospective studies have reported resistance to crizotinib driven by one of these bypass-signaling pathways in 42–44% of crizotinib-resistant ROS1-rearranged NSCLC tumors (31,39,72).
In a series of 75 patients with ROS1 rearranged NSCLCs treated with ROS1 inhibitors, eight (11%) were shown to have concurrent MAPK alterations. Increased HER2 phosphorylation has been identified in a crizotinib- resistant CD74-ROS1 NSCLC patient (73). KRAS G12D, BRAF V600E or KIT D816G mutations have been detected in the clinical setting after progression to crizotinib (10,74-76). EGFR mutations rarely co- occur with ROS1 fusions in the clinical setting (77,78). However, EGFR pathway activation is a relevant mechanism of resistance to ROS1 inhibition (54,79,80).
Combination therapies to overcome this kind of off-target resistance is a promising strategy already tested preclinically (81), although clinical responses have yet to be reported. In addition, it may be challenging to achieve maximum drug levels for each drug in combination therapy due to the potential additive side effects or resulting in too toxic combinations (10). Novel treatment methods such as multiple low dose therapy (MLD) and adaptive dose escalation can be an effective strategy to overcome TKI-resistant NSCLC tumors without significantly increasing toxicity, with encouraging results (82,83).
Phenotypical changes
Phenotypic changes, like epithelial to mesenchymal transition (EMT), can emerge as a resistance mechanism to crizotinib. In one patient with crizotinib-resistant, ROS1-fusion positive NSCLC, no alterations were identified in ROS1, ALK, MET, EGFR, or KRAS, but EMT-like alterations (reduced E-cadherin and increased vimentin) was reported as the potential acquired resistance mechanism (8,79).
The most extreme phenotypic change is the histologic transformation from adenocarcinoma to small-cell lung cancer (SCLC), a known phenomenon in TKI acquired resistance in EGFR-mutant NSCLC (8,84). Recently, Lin et al. reported histologic transformation to SCLC in TKI-resistant ROS1+ NSCLC (84). Currently, these patients should receive standard platinum-etoposide based chemotherapy and be treated like a SCLC, as ROS1 dependance has not been proven for these tumors. Increased knowledge on the fundaments of phenotypic changes in lung adenocarcinoma harboring ROS1 fusions can be critical for the development of more accurate targeted therapies.
Other agents targeting ROS1—first line
Although crizotinib has been so far the standard-of care for patients with ROS1-rearranged NSCLC, other TKI have been tested in the first-line. Resistance mechanism to different TKIs used in the front-line treatment other than crizotinib for ROS1-positive cancer patients can modified subsequent therapies.
Entrectinib
Entrectinib is a multikinase inhibitor targeting TRK, ROS1 and ALK. Notably, entrectinib can effectively cross the blood-brain barrier (85,86) acting on preexisting CNS lesions and preventing or delaying the onset of metastases to the brain (87). However, entrectinib has little to no role in treating crizotinib-resistant ROS1-positive NSCLC. The results of three large multicenter trials (the phase I ALKA-372-001 trial, NCT02097810; the phase I STARTRK-1 study, NCT02097810; and the phase II STARTRK-2 trial; NCT02568267) for entrectinib in ROS1-positive NSCLC were reported in 2018 leading to FDA approval for the first-line treatment of advanced ROS1-rearranged lung cancer.
Fifty-three ROS1-rearranged and ROS1-inhibitor-naïve NSCLC patients were evaluated for entrectinib with an ORR of 77%, an intracranial ORR of 74%, a median duration of response (mDOR) of 24.6 months, and median PFS of 19 months (86). Outcomes were similar to those reported with crizotinib, despite the much higher proportion of patients with baseline CNS metastases in entrectinib trials (>40% vs. 18%, respectively). Although cross-trial indirect comparisons should be avoided, and no face to face comparative trials are expected, entrectinib might be a better option than crizotinib in those patients with CNS metastases at diagnosis and could potentially delay the development of new brain metastases in those without intracranial disease (10).
Substitutions engendering resistance to entrectinib include F2004C/I and G2032R (10).
Entrectinib has no activity against the ROS1 resistance mutations L2026M, G2032R, and D2033N (86,87) and seems to be ineffective in treating crizotinib resistant ROS1-rearranged tumors (8,30).
Ceritinib
Ceritinib is a strong and selective ALK and ROS1 inhibitor with efficacy in ROS1-rearranged NSCLC and BBB penetration (88). However, its use is limited by the lack of regulatory approval in this setting and severe gastrointestinal toxicities (diarrhea, nausea, anorexia, and vomiting) at the recommended full dose (10).
In a phase II study, 30 patients with crizotinib-naïve ROS1-positive metastatic lung cancer were treated with ceritinib, showing an ORR of 62%, a disease control rate (DCR) of 81% and a median PFS of 19.3 month. In eight patients with CNS metastases, the intracranial ORR was 25% with an intracranial DCR of 63%. No responses in the two crizotinib-resistant patients were reported.
Ceritinib shows no activity against most ROS1 resistant mutations, including ROS1 G2032R, so its use is limited to crizotinib-naïve patients. ROS1 E1990G and F1994L substitutions have been reported as resistance mechanism to ceritinib (10).
Brigatinib
Brigatinib is approved in metastatic ALK-fusion positive lung cancer and, like crizotinib and ceritinib, it also has activity against ROS1-fusion tumors based on preclinical studies (8). A phase 1/2 study of brigatinib included 3 patients with ROS1-rearranged NSCLC. One patient was crizotinib- naïve and had a partial response. Of the two crizotinib-pretreated patients, one had stable disease and the other developed progressive disease. The activity of brigatinib against acquired ROS1 mutations seems to be comparable to ceritinib, based on Ba/F3 models. In vitro, brigatinib inhibits the L2026M mutation, but not other common resistance mutations as G2032R, L1951R, D2033N. Therefore, it likely has limited activity against crizotinib-resistant ROS1-driven tumors. Toxicity related to brigatinib includes nausea, diarrhea, headache, and cough. Additionally, it has been associated with early pulmonary toxicity including with pneumonitis, so a two-step dosing has been recommended (8).
Overcoming crizotinib-resistance in ROS1-rearranged NSCLC
Other treatment strategies are available upon crizotinib progression and include the use of chemotherapy, immunotherapy or targeted therapies (Figure 2). Targeted therapies against ROS1 kinase domain can be selective (type I) or multikinase inhibitors (type II) with off-target effects. In some cases, solitary progressions can be managed with local ablative treatments to prolong the benefit of crizotinib. The use of selective treatments based on the mechanism of resistance remains experimental and the indication of rebiopsy either by tissue biopsy or liquid biopsy is not currently validated and recommended outside of clinical trials.
Liquid biopsies in NSCLC
Liquid biopsies are simple, non-invasive blood tests to detect circulating tumor DNA (ctDNA) and have been shown to be non-inferior to up-front tumor tissue genotyping in NSCLC (89). Prior clinical data have demonstrated the sensitivity of plasma ctDNA testing for the detection of common driver mutations in NSCLC, including ROS1 fusions (89-92). A recent review by Drilon et colleagues brought up this unanswered clinical question for discussion (10).
After progression to first-line targeted TKI, a wide spectrum of molecular changes and resistance mechanism can arise. Tissue biopsy can be difficult to obtain in some cases (due to initial response, intracranial disease, oligoprogression) and intratumor heterogeneity must be also taken into account (10). Genomic heterogeneity directly impacts therapeutic choices; depending on the type of the resistance mechanisms in an individual patient, different treatments should be considered.
Analysis of ctDNA can show an integrative view of molecular alterations that can be missed by only tissue biopsy. The non-invasive nature of liquid biopsy allows serial monitoring for acquired resistance mechanisms. However, liquid biopsies have also limitations, as may be influenced by the low ctDNA shedding by the tumor or low allelic fraction, for example in low-tumor burden or solitary brain metastasis, but sensitivity can vary depending on the detecting methodology. In addition, off-target resistance by bypassing signaling or phenotypical changes are also missed in liquid biopsies based on ctDNA. The clinical implications of liquid biopsy findings for the optimal selection of therapy, particularly before radiographic progression, remain to be prospectively assessed (10).
Serial tumor biopsy
Given the rarity of ROS1-rearranged NSCLC and the limited preclinical models or ROS1 fusion lung cancer there is a collective effort to analyze tissue and generate cell lines or patient-derived xenografts (PDX). This is of particular interest in the case of acquired resistance to ROS1 inhibitors as crizotinib. In this regard, the ROS1 Cancer Model Project aims to generate valid research models from patients to understand ROS1 biology, resistance mechanisms, and biomarker testing (93).
Second and subsequent lines of therapy in ROS1-rearranged NSCLC
Crizotinib is highly effective in patients with ROS1 TKI- naive disease, but most tumors invariably acquire one of the resistance mechanisms previously reviewed, limiting their long-term clinical benefit. Additionally, intracranial activity is poor. Therefore, novel agents have been developed to overcome crizotinib resistance and CNS progression disease. Next-generation TKIs can be clinically active against ROS1-rearranged NSCLC after progression to crizotinib, depending on the acquired resistance mechanism, or have substantial intracranial activity compared to crizotinib (10).
Lorlatinib
Lorlatinib (PF-06463922) is an elegantly designed, highly potent small-molecule oral TKI designed for selective ALK/ROS1 inhibition, and robust CNS penetration (94).
In patients treated at the standard 100 mg daily dosing, lorlatinib had a cerebrospinal fluid to plasma ratio ranging 61–96%, indicating an excellent CNS penetration (59,95). Notably, lorlatinib has in vitro activity against several crizotinib-resistant mutations, including L2026M (64), S1986Y/F (96), and D2033N (66).
In a phase II trial of ROS1-rearranged NSCLC patients, 70% of which had progressed to crizotinib, lorlatinib achieved an ORR of 36.2%, intracranial ORR of 56%, and a median PFS of 9.6 months (94). Lorlatinib was overall well tolerated, and the most frequent adverse events were hypercholesterolemia (72%), hypertriglyceridemia (39%), peripheral neuropathy (39%), and peripheral edema (39%) (94).
However, lorlatinib has limited efficacy against the ROS1 G2032R in preclinical models. Thus, lorlatinib could be clinically active in selected patients after crizotinib but may have limited efficacy in the subset of patients with tumors known to harbor G2032R (21).
Although lorlatinib has been recommended by the NCCN Guidelines for the treatment of advanced ROS1-rearranged NSCLC that progressed after crizotinib, entrectinib or ceritinib, the approval by the US FDA and EMA in this indication is still pending.
Given its higher intracranial ORR, lorlatinib can reestablish durable disease control in ROS1-rearranged cancers with pharmacokinetic intracranial failure and/or a limited spectrum of resistance mutations (10). However, its activity against the ROS1 G2032R mutation might be limited in the clinic; Based on preclinical studies, G2032R appears to significantly decrease the cellular potency of lorlatinib. Performing biomarker studies with tissue or blood-based NGS test after progression to crizotinib could identify patients who would benefit more from this treatment.
Repotrectinib
Repotrectinib (TPX-0005) is a potent ALK/ROS1/TRK inhibitor that has shown promising clinical activity in patients with ROS1-rearranged NSCLC and in patients with acquired resistant mutations resistant to prior TKI (30). Repotrectinib is active in CNS and against several ROS1- point resistance mechanisms, including ROS1 G2032R (65,97).
The phase I/II trial (TRIDENT-1, NCT03093116) assessing repotrectinib enrolled 11 TKI-naïve ROS1-rearranged NSCLC patients, showing an ORR of 82%. In 18 TKI pre-treated patients, ORR was 57% at the recommended dose (60). The intracranial ORR was 75%. The responders included patients who received two or more prior TKIs and had tumors harboring the ROS1 G2032R resistance mutation with an ORR 43%, and disease regression occurred in all patients.
Repotrectinib is active both intracranially and against several ROS1- intrinsic resistance mechanisms, including ROS1 G2032R (65). Based in these results, repotrectinib has been granted FDA Fast Track Designation in patients with ROS1-rearranged NSCLC who have progressed on one ROS1 TKI and a platinum-doublet chemotherapy (10).
Taletrectinib
Taletrectinib (DS-6051b) is an oral, small molecule TKI with high affinity for ROS1 and NTRK kinases. In preclinical models, taletrectinib overcomes resistance to ROS1 G2032R mutation (98). In a phase 1 trial (63) taletrectinib showed an ORR of 33% in crizotinib-resistant patients. Regrettably, ROS1 mutation status was not determined, so the efficacy of this agent in the setting of ROS1 on-target resistance is yet unknown (10).
The most common treatment-related AEs were transaminitis (80%), diarrhea (53%), nausea (46%), and constipation (33%) (63).
Cabozantinib
Cabozantinib is a multi-kinase inhibitor with activity against RET, MET, VEGFR2, ALX, TIE2, KIT, and ROS1 (66). Cabozantinib is already approved for use in medullary thyroid cancer and advanced renal cell carcinoma after prior anti-angiogenic therapy. Moreover, cabozantinib has also demonstrated clinical activity against ROS1 fusions, particularly against solvent front resistance mutations in ROS1 including G2032R and D2033N (99).
Within a phase II study, cabozantinib achieved a major partial response in a patient with CD74-ROS1-rearranged NSCLC, who had progressed on crizotinib with an secondary solvent-front D2033N mutation (66). Moreover, a case series of four patients with crizotinib and ceritinib-resistant ROS1-rearranged NSCLC reported by Sun et al. showed 1 partial response and 3 stable disease, including patients with intracranial response, with PFS durations ranged from 4.9 to 13.8 months (61).
Cabozantinib as a multi-targeted TKI, and due to its lack of selectivity, causes significant off-target toxicity not observed with more selective ROS1 TKIs, including gastrointestinal toxicities (diarrhea), cardiovascular toxicities such as hypertension, and palmar-plantar erythrodysesthesia. As a consequence, most patients require dose reductions because of severe adverse events (8).
The spectrum of acquired resistance to type II TKI can differ from type I TKIs. Substitutions observed with type II ROS1 TKIs as cabozantinib include E1974K, F2004V/C, E2020K, V2089M, D2113N/G, M2134I, and F2075V none of which involves solvent- front or gatekeeper residues (10).
Chemo-immunotherapy
After the failure of targeted therapies, conventional cytotoxic chemotherapy remains a standard treatment. Several studies have shown that pemetrexed-based chemotherapy for ROS1 fusion-positive tumors is associated with better responses rates and longer PFS compared with patients with NSCLC harboring other driver mutations (100).
Finally, although prior data supports the lack of response to checkpoint blockade in actionable mutation-driven NSCLC, several case reports have demonstrated the potential role of ICIs either alone or in synergistic combination in ROS1-rearranged NSCLC (101,102).
Conclusion
The development of targeted therapies against driver mutations have dramatically improved the prognosis of lung cancer patients harboring these actionable mutations, including patients with ROS1-rearranged NSCLC.
At this time, several major resistance mechanisms have arisen to oral TKI that lead to treatment failure and next-generation TKIs have been developed in order to overcome resistances. Intracranial activity, efficacy against resistant ROS1-mutant kinases (particularly G2032R), and testing combination therapies for bypass-signaling resistance mechanisms may be keys for prolonging disease control and improving survival.
Tissue or blood-based NGS can help to identify the resistance mechanism to ROS1 TKI and may be considered after tumor progression in order to better select further lines of targeted therapy although prospective validation of this therapeutic approach is needed. In the precision medicine era, a longitudinal assessment of the mechanism of resistance on an individual patient basis may unravel the best treatment strategy and sequence.
Acknowledgments
We thank Dr. Ainhoa Madariaga for article editing and first review.
Funding: None.
Footnote
Provenance and Peer Review: This article was commissioned by the Guest Editors (Jesús Corral, Laura Mezquita and Ernest Nadal) for the series “ALK and ROS-1 NSCLC Patients Treatment Approach Based on Genomic Profile by Liquid Biopsy” published in Precision Cancer Medicine. The article has undergone external peer review.
Conflicts of interest: Both authors have completed the ICMJE uniform disclosure form (available at http://dx.doi.org/10.21037/pcm-20-65). The series “ALK and ROS-1 NSCLC Patients Treatment Approach Based on Genomic Profile by Liquid Biopsy” was commissioned by the editorial office without any funding or sponsorship. Dr. AC reports personal fees from AstraZeneca, personal fees from Boehringer-Ingelheim, personal fees from Pfizer, personal fees from Roche/Genentech, personal fees from Eli Lilly, personal fees from Takeda, personal fees from Novartis, personal fees from Merck Sharp & Dohme, personal fees from Bristol-Myers Squibb, outside the submitted work. Both authors have no other conflicts of interest to declare.
Ethical Statement: Both authors are accountable for all aspects of the work in ensuring that questions related to the accuracy or integrity of any part of the work are appropriately investigated and resolved.
Open Access Statement: This is an Open Access article distributed in accordance with the Creative Commons Attribution-NonCommercial-NoDerivs 4.0 International License (CC BY-NC-ND 4.0), which perdmits the non-commercial replication and distribution of the article with the strict proviso that no changes or edits are made and the original work is properly cited (including links to both the formal publication through the relevant DOI and the license). See: https://creativecommons.org/licenses/by-nc-nd/4.0/.
References
- Shibuya M, Hanafusa H, Balduzzi PC. Cellular sequences related to three new onc genes of avian sarcoma virus (fps, yes, and ros) and their expression in normal and transformed cells. J Virol 1982;42:143-52. [Crossref] [PubMed]
- Matsushime H, Wang LH, Shibuya M. Human c-ros-1 gene homologous to the v-ros sequence of UR2 sarcoma virus encodes for a transmembrane receptorlike molecule. Mol Cell Biol 1986;6:3000-4. [Crossref] [PubMed]
- Birchmeier C, Birnbaum D, Waitches G, et al. Characterization of an activated human ros gene. Mol Cell Biol 1986;6:3109-16. [Crossref] [PubMed]
- Nagarajan L, Louie E, Tsujimoto Y, et al. The human c-ros gene (ROS) is located at chromosome region 6q16----6q22. Proc Natl Acad Sci U S A 1986;83:6568-72. [Crossref] [PubMed]
- Satoh H, Yoshida MC, Matsushime H, et al. Regional localization of the human c-ros-1 on 6q22 and flt on 13q12. Jpn J Cancer Res GANN 1987;78:772-5. [PubMed]
- Acquaviva J, Wong R, Charest A. The multifaceted roles of the receptor tyrosine kinase ROS in development and cancer. Biochim Biophys Acta 2009;1795:37-52. [PubMed]
- Robinson DR, Wu YM, Lin SF. The protein tyrosine kinase family of the human genome. Oncogene 2000;19:5548-57. [Crossref] [PubMed]
- Lin JJ, Shaw AT. Recent Advances in Targeting ROS1 in Lung Cancer. J Thorac Oncol 2017;12:1611-25. [Crossref] [PubMed]
- Sonnenberg-Riethmacher E, Walter B, Riethmacher D, et al. The c-ros tyrosine kinase receptor controls regionalization and differentiation of epithelial cells in the epididymis. Genes Dev 1996;10:1184-93. [Crossref] [PubMed]
- Drilon A, Jenkins C, Iyer S, et al. ROS1-dependent cancers - biology, diagnostics and therapeutics. Nat Rev Clin Oncol 2021;18:35-55. [Crossref] [PubMed]
- Kiyozumi D, Noda T, Yamaguchi R, et al. NELL2-mediated lumicrine signaling through OVCH2 is required for male fertility. Science 2020;368:1132-5. [Crossref] [PubMed]
- Birchmeier C, Sharma S, Wigler M. Expression and rearrangement of the ROS1 gene in human glioblastoma cells. Proc Natl Acad Sci U S A 1987;84:9270-4. [Crossref] [PubMed]
- Aisner DL, Nguyen TT, Paskulin DD, et al. ROS1 and ALK fusions in colorectal cancer, with evidence of intratumoral heterogeneity for molecular drivers. Mol Cancer Res MCR 2014;12:111-8. [Crossref] [PubMed]
- Birch AH, Arcand SL, Oros KK, et al. Chromosome 3 anomalies investigated by genome wide SNP analysis of benign, low malignant potential and low grade ovarian serous tumours. PloS One 2011;6:e28250. [Crossref] [PubMed]
- Gu TL, Deng X, Huang F, et al. Survey of tyrosine kinase signaling reveals ROS kinase fusions in human cholangiocarcinoma. PloS One 2011;6:e15640. [Crossref] [PubMed]
- Davies KD, Doebele RC. Molecular pathways: ROS1 fusion proteins in cancer. Clin Cancer Res 2013;19:4040-5. [Crossref] [PubMed]
- Yamamoto H, Yoshida A, Taguchi K, et al. ALK, ROS1 and NTRK3 gene rearrangements in inflammatory myofibroblastic tumours. Histopathology 2016;69:72-83. [Crossref] [PubMed]
- Lee J, Lee SE, Kang SY, et al. Identification of ROS1 rearrangement in gastric adenocarcinoma. Cancer 2013;119:1627-35. [Crossref] [PubMed]
- Giacomini CP, Sun S, Varma S, et al. Breakpoint analysis of transcriptional and genomic profiles uncovers novel gene fusions spanning multiple human cancer types. PLoS Genet 2013;9:e1003464. [Crossref] [PubMed]
- Roskoski R. ROS1 protein-tyrosine kinase inhibitors in the treatment of ROS1 fusion protein-driven non-small cell lung cancers. Pharmacol Res 2017;121:202-12. [Crossref] [PubMed]
- Davies KD, Le AT, Theodoro MF, et al. Identifying and targeting ROS1 gene fusions in non-small cell lung cancer. Clin Cancer Res 2012;18:4570-9. [Crossref] [PubMed]
- Charest A, Wilker EW, McLaughlin ME, et al. ROS fusion tyrosine kinase activates a SH2 domain-containing phosphatase-2/phosphatidylinositol 3-kinase/mammalian target of rapamycin signaling axis to form glioblastoma in mice. Cancer Res 2006;66:7473-81. [Crossref] [PubMed]
- Jun HJ, Johnson H, Bronson RT, et al. The oncogenic lung cancer fusion kinase CD74-ROS activates a novel invasiveness pathway through E-Syt1 phosphorylation. Cancer Res 2012;72:3764-74. [Crossref] [PubMed]
- Sehgal K, Patell R, Rangachari D, et al. Targeting ROS1 rearrangements in non-small cell lung cancer with crizotinib and other kinase inhibitors. Transl Cancer Res 2018;7:S779-86. [Crossref] [PubMed]
- Rikova K, Guo A, Zeng Q, et al. Global survey of phosphotyrosine signaling identifies oncogenic kinases in lung cancer. Cell 2007;131:1190-203. [Crossref] [PubMed]
- Bergethon K, Shaw AT, Ou SHI, et al. ROS1 rearrangements define a unique molecular class of lung cancers. J Clin Oncol 2012;30:863-70. [Crossref] [PubMed]
- Gainor JF, Shaw AT. Novel targets in non-small cell lung cancer: ROS1 and RET fusions. Oncologist 2013;18:865-75. [Crossref] [PubMed]
- Takeuchi K, Soda M, Togashi Y, et al. RET, ROS1 and ALK fusions in lung cancer. Nat Med 2012;18:378-81. [Crossref] [PubMed]
- Li Z, Shen L, Ding D, et al. Efficacy of Crizotinib among Different Types of ROS1 Fusion Partners in Patients with ROS1-Rearranged Non–Small Cell Lung Cancer. J Thorac Oncol 2018;13:987-95. [Crossref] [PubMed]
- Guo Y, Cao R, Zhang X, et al. Recent Progress in Rare Oncogenic Drivers and Targeted Therapy for Non-Small Cell Lung Cancer. Onco Targets Ther 2019;12:10343-60. [Crossref] [PubMed]
- Gainor JF, Tseng D, Yoda S, et al. Patterns of Metastatic Spread and Mechanisms of Resistance to Crizotinib in ROS1-Positive Non-Small-Cell Lung Cancer. JCO Precis Oncol 2017;2017:PO.17.00063.
- Chiari R, Ricciuti B, Landi L, et al. ROS1-rearranged Non-small-cell Lung Cancer is Associated With a High Rate of Venous Thromboembolism: Analysis From a Phase II, Prospective, Multicenter, Two-arms Trial (METROS). Clin Lung Cancer 2020;21:15-20. [Crossref] [PubMed]
- Ng TL, Smith DE, Mushtaq R, et al. ROS1 Gene Rearrangements Are Associated With an Elevated Risk of Peridiagnosis Thromboembolic Events. J Thorac Oncol 2019;14:596-605. [Crossref] [PubMed]
- Lin JJ, Ritterhouse LL, Ali SM, et al. ROS1 Fusions Rarely Overlap with Other Oncogenic Drivers in Non-Small Cell Lung Cancer. J Thorac Oncol 2017;12:872-7. [Crossref] [PubMed]
- Zeng L, Yang N, Zhang Y. GOPC-ROS1 Rearrangement as an Acquired Resistance Mechanism to Osimertinib and Responding to Crizotinib Combined Treatments in Lung Adenocarcinoma. J Thorac Oncol 2018;13:e114-6. [Crossref] [PubMed]
- Lindeman NI, Cagle PT, Aisner DL, et al. Updated Molecular Testing Guideline for the Selection of Lung Cancer Patients for Treatment With Targeted Tyrosine Kinase Inhibitors: Guideline From the College of American Pathologists, the International Association for the Study of Lung Cancer, and the Association for Molecular Pathology. Arch Pathol Lab Med 2018;142:321-46. [Crossref] [PubMed]
- Garrido P, Conde E, de Castro J, et al. Updated guidelines for predictive biomarker testing in advanced non-small-cell lung cancer: a National Consensus of the Spanish Society of Pathology and the Spanish Society of Medical Oncology. Clin Transl Oncol 2020;22:989-1003. [Crossref] [PubMed]
- NCCN Clinical Practice Guidelines in Oncology (NCCN Guidelines). Non-small cell lung cancer. Version 6.2020. Available online: www.nccn.org. Published 2017. Accessed July 20, 2020.
- Morris TA, Khoo C, Solomon BJ. Targeting ROS1 Rearrangements in Non-small Cell Lung Cancer: Crizotinib and Newer Generation Tyrosine Kinase Inhibitors. Drugs 2019;79:1277-86. [Crossref] [PubMed]
- Davies KD, Le AT, Sheren J, et al. Comparison of Molecular Testing Modalities for Detection of ROS1 Rearrangements in a Cohort of Positive Patient Samples. J Thorac Oncol 2018;13:1474-82. [Crossref] [PubMed]
- Asao T, Takahashi F, Takahashi K. Resistance to molecularly targeted therapy in non-small-cell lung cancer. Respir Investig 2019;57:20-6. [Crossref] [PubMed]
- Shaw AT, Camidge DR, Engelman JA, et al. Clinical activity of crizotinib in advanced non-small cell lung cancer (NSCLC) harboring ROS1 gene rearrangement. J Clin Oncol 2012;30:7508. [Crossref]
- Ou SHI, Bang YJ, Camidge DR, et al. Efficacy and safety of crizotinib in patients with advanced ROS1-rearranged non-small cell lung cancer (NSCLC). J Clin Oncol 2013;31:8032. [Crossref]
- Yasuda H, de Figueiredo-Pontes LL, et al. Preclinical rationale for use of the clinically available multitargeted tyrosine kinase inhibitor crizotinib in ROS1-translocated lung cancer. J Thorac Oncol 2012;7:1086-90. [Crossref] [PubMed]
- Ou SHI, Tan J, Yen Y, et al. ROS1 as a ‘druggable’ receptor tyrosine kinase: lessons learned from inhibiting the ALK pathway. Expert Rev Anticancer Ther 2012;12:447-56. [Crossref] [PubMed]
- Rotow J, Bivona TG. Understanding and targeting resistance mechanisms in NSCLC. Nat Rev Cancer 2017;17:637-58. [Crossref] [PubMed]
- Shaw AT, Riely GJ, Bang YJ, et al. Crizotinib in ROS1-rearranged advanced non-small-cell lung cancer (NSCLC): updated results, including overall survival, from PROFILE 1001. Ann Oncol 2019;30:1121-6. [Crossref] [PubMed]
- Planchard D, Popat S, Kerr K, et al. Metastatic non-small cell lung cancer: ESMO Clinical Practice Guidelines for diagnosis, treatment and follow-up. Ann Oncol 2018;29:iv192-237. [Crossref]
- Mazières J, Zalcman G, Crinò L, et al. Crizotinib therapy for advanced lung adenocarcinoma and a ROS1 rearrangement: results from the EUROS1 cohort. J Clin Oncol 2015;33:992-9. [Crossref] [PubMed]
- Moro-Sibilot D, Faivre L, Zalcman G, et al. Crizotinib in patients with advanced ROS1-rearranged non-small cell lung cancer (NSCLC). Preliminary results of the ACSé phase II trial. J Clin Oncol 2015;33:8065. [Crossref]
- Wu YL, Yang JCH, Kim DW, et al. Phase II Study of Crizotinib in East Asian Patients With ROS1-Positive Advanced Non-Small-Cell Lung Cancer. J Clin Oncol 2018;36:1405-11. [Crossref] [PubMed]
- Facchinetti F, Loriot Y, Kuo MS, et al. Crizotinib-Resistant ROS1 Mutations Reveal a Predictive Kinase Inhibitor Sensitivity Model for ROS1- and ALK-Rearranged Lung Cancers. Clin Cancer Res 2016;22:5983-91. [Crossref] [PubMed]
- McCoach CE, Le AT, Gowan K, et al. Resistance Mechanisms to Targeted Therapies in ROS1+ and ALK+ Non-small Cell Lung Cancer. Clin Cancer Res 2018;24:3334-47. [Crossref] [PubMed]
- Davies KD, Mahale S, Astling DP, et al. Resistance to ROS1 inhibition mediated by EGFR pathway activation in non-small cell lung cancer. PLoS One 2013;8:e82236. [Crossref] [PubMed]
- Costa DB, Kobayashi S, Pandya SS, et al. CSF concentration of the anaplastic lymphoma kinase inhibitor crizotinib. J Clin Oncol 2011;29:e443-5. [Crossref] [PubMed]
- Okimoto T, Tsubata Y, Hotta T, et al. A Low Crizotinib Concentration in the Cerebrospinal Fluid Causes Ineffective Treatment of Anaplastic Lymphoma Kinase-positive Non-small Cell Lung Cancer with Carcinomatous Meningitis. Intern Med 2019;58:703-5. [Crossref] [PubMed]
- Ou SHI, Zhu VW. CNS metastasis in ROS1+ NSCLC: An urgent call to action, to understand, and to overcome. Lung Cancer 2019;130:201-7. [Crossref] [PubMed]
- Drilon A, Siena S, Dziadziuszko R, et al. Entrectinib in ROS1 fusion-positive non-small-cell lung cancer: integrated analysis of three phase 1-2 trials. Lancet Oncol 2020;21:261-70. [Crossref] [PubMed]
- Shaw AT, Solomon BJ, Chiari R, et al. Lorlatinib in advanced ROS1-positive non-small-cell lung cancer: a multicentre, open-label, single-arm, phase 1-2 trial. Lancet Oncol 2019;20:1691-701. [Crossref] [PubMed]
- Safety and preliminary clinical activity of repotrectinib in patients with advanced ROS1 fusion-positive non-small cell lung cancer (TRIDENT-1 study). Journal of Clinical Oncology [Internet]. [cited 2020 Sep 25]. Available online: https://ascopubs.org/doi/abs/10.1200/JCO.2019.37.15_suppl.9011
- Sun TY, Niu X, Chakraborty A, et al. Lengthy Progression-Free Survival and Intracranial Activity of Cabozantinib in Patients with Crizotinib and Ceritinib-Resistant ROS1-Positive Non-Small Cell Lung Cancer. J Thorac Oncol 2019;14:e21-4. [Crossref] [PubMed]
- Drilon A, Ou SHI, Cho BC, et al. Repotrectinib (TPX-0005) Is a Next-Generation ROS1/TRK/ALK Inhibitor That Potently Inhibits ROS1/TRK/ALK Solvent- Front Mutations. Cancer Discov 2018;8:1227-36. [Crossref] [PubMed]
- Fujiwara Y, Takeda M, Yamamoto N, et al. Safety and pharmacokinetics of DS-6051b in Japanese patients with non-small cell lung cancer harboring ROS1 fusions: a phase I study. Oncotarget 2018;9:23729-37. [Crossref] [PubMed]
- Zou HY, Li Q, Engstrom LD, et al. PF-06463922 is a potent and selective next-generation ROS1/ALK inhibitor capable of blocking crizotinib-resistant ROS1 mutations. Proc Natl Acad Sci U S A 2015;112:3493-8. [Crossref] [PubMed]
- Cui JJ, Zhai D, Deng W, et al. TPX-0005, a novel ALK/ROS1/TRK inhibitor, effectively inhibited a broad spectrum of mutations including solvent front ALK G1202R, ROS1 G2032R and TRKA G595R mutants. Eur J Cancer 2016;69:S32. [Crossref]
- Drilon A, Somwar R, Wagner JP, et al. A Novel Crizotinib-Resistant Solvent-Front Mutation Responsive to Cabozantinib Therapy in a Patient with ROS1-Rearranged Lung Cancer. Clin Cancer Res 2016;22:2351-8. [Crossref] [PubMed]
- Gong B, Oh-Hara T, Fujita N, et al. 3D culture system containing gellan gum restores oncogene dependence in ROS1 rearrangements non-small cell lung cancer. Biochem Biophys Res Commun 2018;501:527-33. [Crossref] [PubMed]
- Chong CR, Bahcall M, Capelletti M, et al. Identification of Existing Drugs That Effectively Target NTRK1 and ROS1 Rearrangements in Lung Cancer. Clin Cancer Res 2017;23:204-13. [Crossref] [PubMed]
- Abstract 1319: Repotrectinib, a new generation ROS1 inhibitor, is highly potent against fusion ROS1s and emerging resistance mutations | Cancer Research [Internet]. [cited 2021 Apr 1]. Available online: https://cancerres.aacrjournals.org/content/79/13_Supplement/1319
- Roys A, Chang X, Liu Y, et al. Resistance mechanisms and potent-targeted therapies of ROS1-positive lung cancer. Cancer Chemother Pharmacol 2019;84:679-88. [Crossref] [PubMed]
- Awad MM, Engelman JA, Shaw AT. Acquired resistance to crizotinib from a mutation in CD74-ROS1. N Engl J Med 2013;369:1173. [Crossref] [PubMed]
- Gou W, Zhou X, Liu Z, et al. CD74-ROS1 G2032R mutation transcriptionally up-regulates Twist1 in non-small cell lung cancer cells leading to increased migration, invasion, and resistance to crizotinib. Cancer Lett 2018;422:19-28. [Crossref] [PubMed]
- Dagogo-Jack I, Rooney M, Nagy RJ, et al. Molecular Analysis of Plasma From Patients With ROS1-Positive NSCLC. J Thorac Oncol 2019;14:816-24. [Crossref] [PubMed]
- Sato H, Schoenfeld AJ, Siau E, et al. MAPK Pathway Alterations Correlate with Poor Survival and Drive Resistance to Therapy in Patients with Lung Cancers Driven by ROS1 Fusions. Clin Cancer Res 2020;26:2932-45. [Crossref] [PubMed]
- Watanabe J, Furuya N, Fujiwara Y. Appearance of a BRAF Mutation Conferring Resistance to Crizotinib in Non-Small Cell Lung Cancer Harboring Oncogenic ROS1 Fusion. J Thorac Oncol 2018;13:e66-9. [Crossref] [PubMed]
- Zhu YC, Lin XP, Li XF, et al. Concurrent ROS1 gene rearrangement and KRAS mutation in lung adenocarcinoma: A case report and literature review. Thorac Cancer 2018;9:159-63. [Crossref] [PubMed]
- Dziadziuszko R, Le AT, Wrona A, et al. An Activating KIT Mutation Induces Crizotinib Resistance in ROS1-Positive Lung Cancer. J Thorac Oncol 2016;11:1273-81. [Crossref] [PubMed]
- Wiesweg M, Eberhardt WEE, Reis H, et al. High Prevalence of Concomitant Oncogene Mutations in Prospectively Identified Patients with ROS1-Positive Metastatic Lung Cancer. J Thorac Oncol 2017;12:54-64. [Crossref] [PubMed]
- He Y, Sheng W, Hu W, et al. Different Types of ROS1 Fusion Partners Yield Comparable Efficacy to Crizotinib. Oncol Res 2019;27:901-10. [Crossref] [PubMed]
- Song A, Kim TM, Kim DW, et al. Molecular Changes Associated with Acquired Resistance to Crizotinib in ROS1-Rearranged Non–Small Cell Lung Cancer. Clin Cancer Res 2015;21:2379-87. [Crossref] [PubMed]
- Vaishnavi A, Schubert L, Rix U, et al. EGFR Mediates Responses to Small-Molecule Drugs Targeting Oncogenic Fusion Kinases. Cancer Res 2017;77:3551-63. [Crossref] [PubMed]
- Kato Y, Ninomiya K, Ohashi K, et al. Combined effect of cabozantinib and gefitinib in crizotinib-resistant lung tumors harboring ROS1 fusions. Cancer Sci 2018;109:3149-58. [Crossref] [PubMed]
- Fernandes Neto JM, Nadal E, Bosdriesz E, et al. Multiple low dose therapy as an effective strategy to treat EGFR inhibitor-resistant NSCLC tumours. Nat Commun 2020;11:3157. [Crossref] [PubMed]
- Piotrowska Z, Isozaki H, Lennerz JK, et al. Landscape of Acquired Resistance to Osimertinib in EGFR-Mutant NSCLC and Clinical Validation of Combined EGFR and RET Inhibition with Osimertinib and BLU-667 for Acquired RET Fusion. Cancer Discov 2018;8:1529-39. [Crossref] [PubMed]
- Lin JJ, Shaw AT. Resisting Resistance: Targeted Therapies in Lung Cancer. Trends Cancer 2016;2:350-64. [Crossref] [PubMed]
- Menichincheri M, Ardini E, Magnaghi P, et al. Discovery of Entrectinib: A New 3-Aminoindazole As a Potent Anaplastic Lymphoma Kinase (ALK), c-ros Oncogene 1 Kinase (ROS1), and Pan-Tropomyosin Receptor Kinases (Pan-TRKs) inhibitor. J Med Chem 2016;59:3392-408. [Crossref] [PubMed]
- Ardini E, Menichincheri M, Banfi P, et al. Entrectinib, a Pan-TRK, ROS1, and ALK Inhibitor with Activity in Multiple Molecularly Defined Cancer Indications. Mol Cancer Ther 2016;15:628-39. [Crossref] [PubMed]
- Doebele RC, Ahn MJ, Siena S, et al. Efficacy and safety of entrectinib in locally advanced or metastatic ROS1-positive Non-Small Cell Lung Cancer (NSCLC). In Toronto, Canada.; 2018.
- Lim SM, Kim HR, Lee JS, et al. Open-Label, Multicenter, Phase II Study of Ceritinib in Patients With Non-Small-Cell Lung Cancer Harboring ROS1 Rearrangement. J Clin Oncol 2017;35:2613-8. [Crossref] [PubMed]
- Leighl NB, Page RD, Raymond VM, et al. Clinical Utility of Comprehensive Cell-free DNA Analysis to Identify Genomic Biomarkers in Patients with Newly Diagnosed Metastatic Non-small Cell Lung Cancer. Clin Cancer Res 2019;25:4691-700. [Crossref] [PubMed]
- Weber B, Meldgaard P, Hager H, et al. Detection of EGFR mutations in plasma and biopsies from non-small cell lung cancer patients by allele-specific PCR assays. BMC Cancer 2014;14:294. [Crossref] [PubMed]
- Wan JCM, Massie C, Garcia-Corbacho J, et al. Liquid biopsies come of age: towards implementation of circulating tumour DNA. Nat Rev Cancer 2017;17:223-38. [Crossref] [PubMed]
- Sabari JK, Santini F, Bergagnini I, et al. Changing the Therapeutic Landscape in Non-small Cell Lung Cancers: the Evolution of Comprehensive Molecular Profiling Improves Access to Therapy. Curr Oncol Rep 2017;19:24. [Crossref] [PubMed]
- Moore AC, Goldman L, Tomalia T, et al. B16 The ROS1 Cancer Model Project: A Unique Patient-Driven Partnership to Accelerate Research. J Thorac Oncol 2020;15:S32. [Crossref]
- Phase I/II study of PF-06463922, an ALK/ROS1 tyrosine kinase inhibitor, in patients with advanced non-small-cell lung cancer harboring specific molecular alterations. | Journal of Clinical Oncology [Internet]. [cited 2020 Oct 15]. Available online: https://ascopubs.org/doi/abs/10.1200/jco.2015.33.15_suppl.tps2620
- Solomon BJ, Bauer TM, Felip E, et al. Safety and efficacy of lorlatinib (PF-06463922) from the dose-escalation component of a study in patients with advanced ALK+ or ROS1+ non-small cell lung cancer (NSCLC). J Clin Oncol 2016;34:9009. [Crossref]
- Facchinetti F, Rossi G, Bria E, et al. Oncogene addiction in non-small cell lung cancer: Focus on ROS1 inhibition. Cancer Treat Rev 2017;55:83-95. [Crossref] [PubMed]
- Katayama R, Gong B, Togashi N, et al. The new-generation selective ROS1/NTRK inhibitor DS-6051b overcomes crizotinib resistant ROS1-G2032R mutation in preclinical models. Nat Commun 2019;10:3604. [Crossref] [PubMed]
- Katayama R, Kobayashi Y, Friboulet L, et al. Cabozantinib overcomes crizotinib resistance in ROS1 fusion-positive cancer. Clin Cancer Res 2015;21:166-74. [Crossref] [PubMed]
- Chen YF, Hsieh MS, Wu SG, et al. Efficacy of Pemetrexed-Based Chemotherapy in Patients with ROS1 Fusion–Positive Lung Adenocarcinoma Compared with in Patients Harboring Other Driver Mutations in East Asian Populations. J Thorac Oncol 2016;11:1140-52. [Crossref] [PubMed]
- Jain A, Fujioka N, Patel M. Immune Checkpoint Inhibitors in ROS1-Rearranged Non-Small Cell Lung Cancer: A Report of Two Cases. J Thorac Oncol 2019;14:e165-7. [Crossref] [PubMed]
- Calles A, Riess JW, Brahmer JR. Checkpoint Blockade in Lung Cancer With Driver Mutation: Choose the Road Wisely. Am Soc Clin Oncol Educ Book Am Soc Clin Oncol Annu Meet. 2020;40:372-84.
Cite this article as: García-Pardo M, Calles A. ROS-1 NSCLC therapy resistance mechanism. Precis Cancer Med 2021;4:16.